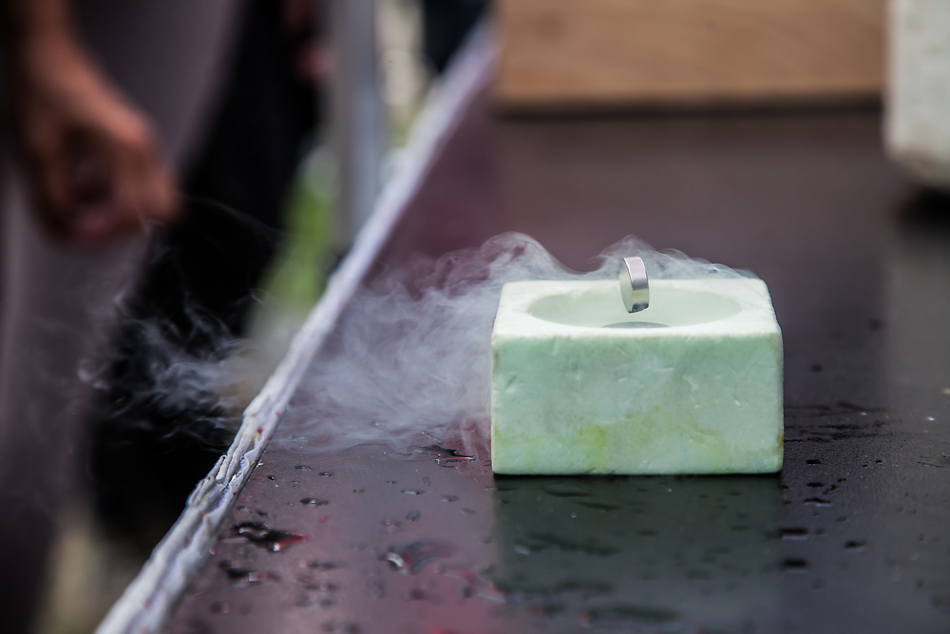
Image Credit: Forance/Shutterstock.com
Superconductivity at room temperature is still an unsolved challenge in science. A superconductor with the capability of operating at ambient temperatures might have the capacity to reduce the energy dissipation by almost 5% during electricity transmission. This would bring a paradigm shift in large-scale energy transmission and energy usage in small-scale computing systems, while it may also work as a reservoir for energy storage. Room-temperature superconductors have the potential to revolutionize the energy industry.
What is a Room-Temperature Superconductor?
Superconductors are a special class of materials that pose zero-resistance electron transmission under a specific threshold temperature typical to the material concerned.
This threshold temperature is called the ‘critical temperature’ or Tc in short. In general, any material can be called a superconductor if it shows the following characteristics below Tc:
- Zero electrical resistance
- Perfect diamagnetism (the Meissner effect)
A room-temperature superconductor can operate at temperatures above 0 ◦C. Even though this is not strictly ‘room temperature’, it is the temperature in which ice forms and is the most easily attainable temperature.
What are Some Examples of Superconducting Materials?
The most promising amongst these materials explored until 2019 is Lanthanum Decahydride (LaH10) with the highest transition temperature recorded at −23 °C/ 250 K.
Hydrogen sulfide was known before this to hold the highest recorded operable temperature of −70 °C/ 203 K. With respect to atmospheric pressure, Cuprate compounds have demonstrated superconductivity at approximately −135 °C/ 138 K. On the other hand, Ternary Superhydrides are predicted to reach very high temperatures of approximately 200 °C/ 473 K, at 250 GPa, which is much higher than atmospheric pressure.
Read more about electrical properties here.
Origins of Room Temperature Superconductivity
The origins of superconductivity were first explained by the Ginzburg-Landau theory. A more precise microscopic explanation of the phenomenon of superconductivity can be derived from the Bardeen, Cooper and Schrieffer (BCS) theory.
The BCS theory has its origin in the concept previously proposed by Cooper that the ground state of a material is unstable with respect to pairs of 'bound' electrons. These ‘bound’ electrons, also called Cooper pairs, are formed by electron-phonon interactions, a phonon being a quanta of crystal lattice vibration.
An electron in the cation lattice distorts the local lattice surrounding it, creating an area of higher positive charge density around itself. Another electron some distance away in the lattice is then attracted to this charge distortion. The electrons become indirectly attracted to each other and mediated by the lattice vibrations, acting as a kind of glue between the two electrons. All this happens at much lower temperatures, usually below Tc.
Find out more about electrical properties characterization equipment.
The reasons behind the resistance-less transmission of Cooper pairs within a superconductor can be explained with respect to two aspects:
- Mathematically speaking, a Cooper pair is more stable than a single electron within the lattice, while it experiences less resistance.
- Physically speaking, the Cooper pair is more resistant to lattice vibrations than a single electron due to the glue that holds the pair of electrons together. They transmit through the lattice relatively unaffected by electron-phonon interactions below Tc.
However, the BCS theory could only predict the theoretical maximum of Tc at around 30-40 K. Above this, the high thermal energy would cause high energy electron-phonon interactions, which might not allow the formation of or sustainment of the Cooper pairs. The mechanism that might explain high-temperature superconductivity is still highly debatable.
Research On Room-Temperature Superconductors: A Chronology
In the absence of a detailed understanding of the mechanism of room-temperature superconductivity, there had only been incremental progress.
The first superconductor, Mercury (Hg) cooled to ultra-low temperature, was discovered by Heike Kamerlingh Onnes, who found that when Hg was placed inside a liquid helium container and cooled to very low temperatures, its electric resistance suddenly dropped to zero.
In 1987, Alex Müller and Georg Bednorz at IBM in Zurich, Switzerland, discovered high-temperature superconductors. These were new ceramic compounds made of copper and oxygen and mixed with other elements such as Lanthanum (La), Barium (Ba) and Bismuth (Bi). Minimal amounts of electrical currents could flow through them.
In more recent times, a group of researchers in Germany’s University of Leipzig, led by Dr. Pablo Esquinazi, have found a 2D graphene structure that shows remarkable electron transmission properties at room temperatures and above.
The team soaked flakes of graphite with water, which induced more free electrons to introduce superconductivity into the structure made out of layers of carbon atoms arranged in hexagonal lattices.
Compounds such as lanthanum superhydrides are considered conventional superconductors, and their superconducting properties have been acknowledged since the 1950s.
In 2018, Russell Hemley and his team from the George Washington University in Washington DC reported observing a sudden drop in electrical resistance at 7 °C in a ‘superhydride’ material synthesized by them. It is a type of compound that contains a large amount of hydrogen along with La (LaH10).
This drop was a sign of superconductivity. They placed a diamond anvil in a synchrotron beamline at the Argonne National Laboratory. They squeezed a minuscule sample of lanthanum and hydrogen using the anvil’s diamond tips by raising pressures to 200 GPa. The compound was then heated, and its structure changed along with its conductive properties. The process was monitored using X-ray diffraction.
They produced a new structure called LaH10, which was previously simulated and studied by the team and others. The structure superconducted at very high temperatures. The material was then cooled while keeping it at high pressure, and its electronic properties were measured.
The electrical resistance was found to drop at a temperature of 7 °C/280 K in some instances. However, in follow-up experiments inside the laboratory, the transition temperature was lower than 7 °C but still an impressive -23 °C.
In 2019, Salvatore Cezar Pais from the US Navy received a patent for an application that showed a room-temperature superconductor could be built using a wire with an insulator core coated with aluminum PZT (Lead Zirconate Titanate) with a thickness of approximately the London penetration depth.
The coating was deposited by vacuum evaporation and was polarized after deposition. He showed that when an electromagnetic coil is positioned circumferentially around the coating, a non-linear vibration is induced, enabling room temperature superconductivity when the coil is activated with a pulsed current.
Neutron Scattering Experiments on Zirconium Vanadium Hydride
In 2020, another international team of researchers with scientists from the Empa research institute (Swiss Federal Laboratories for Materials Science and Technology), the University of Zurich, Polish Academy of Sciences, the University of Illinois at Chicago, and US Department of Energy’s Oak Ridge National Laboratory (ORNL), conducted neutron scattering experiments on Zirconium Vanadium Hydride (ZVH) at atmospheric pressure and at temperatures ranging between 5 K - 250 K at ORNL.
This led to the first observations of extremely small hydrogen-hydrogen atomic distances in the metal hydride ~ 1.6 Angstroms. Its predicted value was 2.1 angstrom.
The hydrogen contained in metals affects their electronic properties and they have been found to start superconducting at very high pressures. A closely spaced (< 2.1 Angstroms) interatomic arrangement of hydrogen is very promising.
The fact that ZVH is an inexpensive, stable metal adds to the potential of this discovery. The team ran computer simulations, along with additional experiments, to prove that the unexpected spectral intensity occurs only when distances between hydrogen atoms are closer than 2.0 Angstroms, something that has never been observed in a metal hydride at ambient pressure and temperature. This was the first known exception to the Switendick criterion in a bimetallic alloy, which states that, for stable Hydrides at standard temperatures and pressures, the H-H distance is never < 2.1 Angstroms.
When researching room-temperature superconductivity, Professor Pegor Aynajian’s group at the Binghamton University, State University of New York, built a Scanning Tunneling Microscope (STM) to help the group to observe the electrons at the surface of the material concerned. This may help to understand how electrons bind (Cooper pairs) and produce superconductivity on an atomic scale.
It has already been long predicted that electrons also pair up in high-temperature superconductors. Therefore, a precise answer to how high-temperature superconductors work might just lead to more precise room-temperature superconductivity.
Superconductivity - the challenge of no resistance at room temperature
Video Credit: Max-Planck-Institut für Chemie/YouTube.com
Future Research and Applications of Room Temperature Superconductivity
Room temperature superconductivity is an elusive and exciting phenomenon, which, if understood and achieved on a large scale, will save billions of dollars in wasted heat for energy transmission. It may have other potential applications such as energy storage, which could power cities and dramatically reduce greenhouse gas emissions.
The scale of potential applications is unimaginable and, therefore, more research needs to be done to understanding how electrons can be glued together at higher temperatures for resistance-less transmission.
References and Further Reading
The University of Bristol. BCS Superconductivity Theory. [Online] Available at: http://www.chm.bris.ac.uk/webprojects2000/igrant/bcstheory.html (Accessed on 23 June 2020).
Aynajian, P. (2020) ROOM-TEMPERATURE SUPERCONDUCTORS COULD REVOLUTIONIZE THE WORLD’S ENERGY [Online] Inverse. Available at: https://www.inverse.com/science/room-temperature-superconductors (Accessed on 23 June 2020).
Scheike, T., Böhlmann, W., Esquinazi, P., Barzola‐Quiquia, J., Ballestar, A. and Setzer, A. (2012) Can Doping Graphite Trigger Room Temperature Superconductivity? Evidence for Granular High‐Temperature Superconductivity in Water‐Treated Graphite Powder. Advanced Materials, 24: 5826-5831. https://doi.org/10.1002/adma.201202219
Carter, T. (2019) Navy files for patent on room-temperature superconductor [Online] Phys.org. Available at: https://phys.org/news/2019-02-navy-patent-room-temperature-superconductor.html (Accessed on 23 June 2020).
Maddury Somayazulu, Muhtar Ahart, Ajay K. Mishra, Zachary M. Geballe, Maria Baldini, Yue Meng, Viktor V. Struzhkin, and Russell J. Hemley. (2019) Evidence for Superconductivity above 260 K in Lanthanum Superhydride at Megabar Pressures. Physical Review Letters. 122, 027001. https://doi.org/10.1103/PhysRevLett.122.027001
Russell J. Hemley .et al. (2020) Inelastic neutron scattering evidence for anomalous H–H distances in metal hydrides. PNAS. 117 (8) 4021-4026. https://doi.org/10.1073/pnas.1912900117
Disclaimer: The views expressed here are those of the author expressed in their private capacity and do not necessarily represent the views of AZoM.com Limited T/A AZoNetwork the owner and operator of this website. This disclaimer forms part of the Terms and conditions of use of this website.