DOI :
10.2240/azojomo0281
Apr 30 2009
Tran Thi Thu Hien, Chanel Ishizaki and Kozo Ishizaki
Copyright AD-TECH; licensee AZoM.com Pty Ltd.
This is an AZo Open Access Rewards System (AZo-OARS) article distributed under the terms of the AZo–OARS https://www.azom.com/oars.asp which permits unrestricted use provided the original work is properly cited but is limited to non-commercial distribution and reproduction.
AZojomo (ISSN 1833-122X) Volume 5 April 200
Topics Covered
Abstract
Keywords
Introduction
Experimental
Binding Energy Calculation
Results and Discussion
Summary and Conclusions
References
Contact Details
Abstract
Seven commercial Si3N4 powders produced by different production processes, nitridation media and further acid treatments were analyzed by XPS technique. The XPS spectra of all as received powders show Si 2p, C 1s, N 1s and O 1s signals. Si 2p signals were deconvoluted using a peak fit software. Tetrahedral structures were observed for all powders with different atoms or groups. The binding energy of the Si-N varies due to differences in the Si nearest neighbor atoms or groups in the tetrahedral. The XPS results show that the main configuration for all powders contains two Si atoms and one N atom in the tetrahedral (Si-SiSiN), the other one atom being OH or NH2 groups except for the powder produced by carbothermal reduction process using NH3(g) as nitridation media the intensity fraction of this configuration is comparable to the Si-SiNNO configuration. For the powders washed by acid as a final treatment (C4 and C6) the main tetrahedral is Si-SiSiN(NH2), for the other powders the main tetrahedral is Si-SiSiN(OH). The results are in good agreement with our previous studies (FTIR) showing that the surface composition is a mixture of tetrahedral units Si-XYZW where X, Y, Z, W are Si, H, N, O atoms or NH2, NH, OH groups.
Keywords
Silicon Nitride; Powder; Surface; Structures; X-ray Photoelectron Spectroscopy (XPS)
Introduction
A major technical issue in the processing of ceramic materials is the sinter-ability of the silicon nitride starting powders. The sinter-ability is determined by the formation of the oxide layers on the surface of the powder particles at sintering temperature. Densification are not directly effected to any kind of Si3N4 powders but to the starting Si3N4 particle surface (for example the amount of silanol surface group) the powder which reveal a sufficiently high number of silanol surface group, leads to a homogeneous distribution of sintering aids [1]. For above reason significant to clarify the state, species, distribution of oxygen present and chemical composition of the surface of the starting Si3N4 powder samples because the nature and amount of sintering aids needed depends upon the extent of oxygen present on the surface. X-ray photoelectron spectroscopy (XPS) is widely used analytical method for investigating the elemental and chemical composition of solid surfaces. Many researchers have been investigated Si3N4 powders surface using different analytical techniques (XPS, ESCA, AES, SIMS...) it was concluded that as-received Si3N4 powders have a surface layer composition similar to an intermediate state between silica and silicon oxynitride [2-6]. Diffuse reflection infrared Fourier transform spectroscopy (DRIFT) has been applied to specify the kinds of surface groups such as SiNH2, Si2NH, SiOH, SiH and SiH2 [7-13]. By Temperature Programmed Desorption Mass Spectroscopy (TPDMS) technique, Nakamatsu et al. [14, 15] found that Si3N4 powders surface are unlike the surface of silica in the kinds and quantities of desorbed molecules. The detected desorbed species were H2, H2O, NH3 and N2 present for all powder but each of them present a unique surface. From the quantitative comparison of the desorbed species up to 1300oC, surface groups on Si3N4 powder surface are strongly affected by the nitridation media, final treatment and production methods. With same Si3N4 powders T. T. T. Hien [16] using FTIR method to analyze surface composition and showed that silicon nitride powder surfaces present tetrahedral units with different atoms (O, N, Si, H) and groups (NH, NH2, OH) configurations, which bond to the same silicon atom. In this paper, Si 2p signal obtained from XPS measurement of seven commercial Si3N4 powders are investigated. >
Experimental
The X-ray photoelectron spectra of Si 2p, C 1s, N 1s and O 1s signals of seven commercial Si3N4 powders, produced by different processes as shown in Table 1, were measured using XPS instrument.
Table 1. Production processes and specifications of the seven commercial silicon nitride (Si3N4) powders.
Nomenclature
|
Oxygen content/mass%
|
Specific surface area/m2g-1
|
Synthesis method
|
Nitridation media
|
A1
|
1.05
|
10.2
|
Silicon diimide precipitation
|
NH3(l)
|
A2
|
0.98
|
9.2
|
NH3(g)
|
B1
|
1.88
|
11.6
|
Carbothermal reduction and nitridation
|
N2
|
B2
|
2.45
|
6.5
|
NH3(g)
|
C4**
|
0.66
|
8.5
|
Nitridation of silicon
|
N2
|
C5*
|
0.83
|
3.9
|
N2 + H2
|
C6**
|
0.78
|
11.6
|
N2 + H2
|
** Crushed and washed by HF acid and water * Crushed
The X-rays used to bombard the sample were monochromatic MgLKα rays with energy of 1253.6 eV. The vacuum in the analysis chamber was 2x10-7 Pa. A narrow scan resolution of 0.1 eV was used. The adventitious C 1s peak, arising from traces of hydrocarbon in the spectrometer, was used as a reference for evaluating the peak positions because of static charging of the sample fixed at B.E = 284.6 eV . The C 1s peak position was observed together with other peaks (Si 2p, N 1s and O 1s) of the spectrum.
Binding Energy Calculation
The Si 2p signal has been reported as the sum of several gaussian curves corresponding to the different silicon-centered tetrahedral [17,18]. The binding energy of Si-N for each tetrahedron was calculated using Miyazaki et al. linear relations [18]. The calculation was not only taken into account Si, C and N atoms as reference but also added H, O atoms or NH, NH2 and OH groups using Pauling’s electro-negativity by considering the coordination number of the respective elements or groups. It was assumed that the proportions of tetrahedral with more than two H atoms or NH, NH2, OH groups were very weak and could be neglected [19]. The results are shown in Table 2 with tetrahedral and binding energy corresponding.
Results and Discussion
The XPS spectra for Si 2p (106 – 96 eV), C 1s (290 – 280 eV), N 1s (402 – 392 eV) and O 1s (538 – 528eV) signals obtained for all powders are shown in Fig 1. The XPS spectra of all as received Si3N4 powders show Si 2p, C 1s, N 1s and O 1s signals but peak intensities and binding energies are different for each powder. The signals seen in Figure 1 have been referenced to the adventitious C1s line [2]. In the Si 2p signal, the positions of the band maxima are a little bit different among the powders. For most of the powders, the maximum is around 101.5 eV but for the two powders washed as further treatment (C4 and C6) it is shifted toward a lower binding energy of about 101.2 eV as seen in the figure. Both of these binding energies have been assigned to Si-N bonds [20, 21]. The Si-N bond binding energy variation is due to differences of silicon near-neighbour atoms as it will be discussed in more details later. The powder produced by nitridation of silicon process using a mixture of N2 + H2 as nitriding media and washed in the further treatment (C6) shows the highest Si 2p and N 1s signals and the lowest O 1s intensity. The maximum of the N1s signals for all the powders is located in the range 397.1 – 397.4 eV, and assigned to N-SiSiSi bonds [18, 22, 23]. The O1s signal is in the range 530.5 – 533.8 eV. Contributions from O-Si, O-C and O-H bonds or even absorbed metal oxide impurities can overlap in this range [17, 23, 24]. It is therefore difficult to separate them because their binding energies are very close. O-N bonds have been reported to have a binding energy in the range 399.7 – 402.8 eV [22, 25]. None of the powders show a considerable peak in this range.
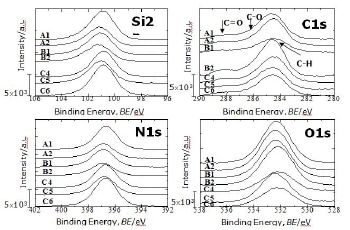
Figure 1. Si 2p, C 1s, N 1s and O 1s signals obtained from XPS measurement
The spectra of Si 2p signal shown in Figure 1 were deconvoluted using a Jandel peak fit and analysis software. Figure 2 shows the fittings with the highest fitting parameters.
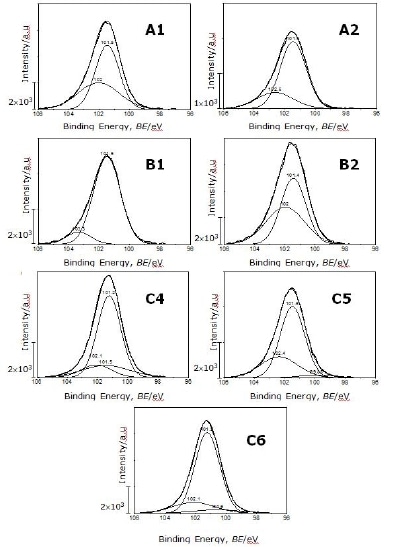
Figure 2. Deconvolution of the Si 2p signal for all Si3N4 powders
The binding energies from the peak separation of the spectra for all powders and possible assignments for tetrahedral configurations are listed in Table 2.
Table 2. Calculated binding energies corresponding configurations The data of configurations that include O and H atoms as well as OH, NH2 and NH groups marked with an * were estimated by the present authors as explained in the text. The ones calculated by Miyazaki et al. are also included [18].
Configurations
|
Binding Energy, BE/eV
|
Si-SiSiSiSi
|
99.4
|
Si-SiSiSiN
|
99.9
|
Si-SiSiSi(NH)*
|
100.4
|
Si-SiSiNN
|
100.5
|
Si-SiSiN(NH)*
|
101
|
Si-SiSiN(NH2)*
|
101.2
|
Si-SiSiN(OH)*
|
101.5
|
Si-NNNN
|
101.7
|
Si-SiNNO*
|
102
|
Si-SiNN(OH)*
|
102.1
|
Si-SiSiHO*
|
102.6
|
Si-NNNO*
|
102.6
|
Si-NNOO*
|
103.4
|
The highest intensity peak obtained for each powder is underlined. For five out of seven powders the highest intensity peak has a binding energy around 101.5 eV can be assigned to a Si-SiSiN(OH) tetrahedral configuration. Exceptions are the two powders produced by direct nitridation of silicon with HF acid washing as final treatment (C4 and C6) where the highest intensity peak has a binding energy of 101.2 eV can be assigned to a Si-SiSiN(NH2) tetrahedral. Only the powder produced by carbothermal reduction process using N2 as nitridation media (B1) shows tetrahedra that includes two O atoms as neighbors of the Si-N bond.
The tetrahedral configurations listed in Table 3 were grouped considering the backbonded atoms or groups as follows: Si-SiSiN(Si; N), Si-SiSiN(NH2), Si-SiSiN(OH), Si-SiNN(OH) and Si-O(SiNN; SiSiH; NNN; NNO).
Table 3. Estimated binding energies from deconvoluted spectra and their assignments for all Si3N4 powders. The highest intensity peaks are underline and correspond to Si-SiSiN(OH) for five powders and Si-SiSiN(NH2) for the two powders produced by nitridation of silicon and washed by acid. The later also differ from there powders by the lack of O containing tetrahedral.
Configurations
|
A1
|
A2
|
B1
|
B2
|
C4
|
C5
|
C6
|
Si-SiSiSiN
|
|
|
|
|
|
99.9
|
|
Si-SiSiNN
|
|
|
|
|
|
|
100.6
|
Si-SiSiN(NH2)
|
|
|
|
|
101.2
|
|
101.2
|
Si-SiSiN(OH)
|
101.5
|
101.5
|
101.5
|
101.4
|
101.5
|
101.5
|
|
Si-SiNNO
|
102
|
|
|
102
|
|
|
|
Si-SiNN(OH)
|
|
|
|
|
102.1
|
|
102.1
|
Si-SiSiHO
|
|
|
|
|
|
102.4
|
|
Si-NNNO
|
|
102.6
|
|
|
|
|
|
Si-NNOO
|
|
|
103.3
|
|
|
|
|
The summation of intensities obtained from the deconvoluted spectra for each group is shown in Figure 3.
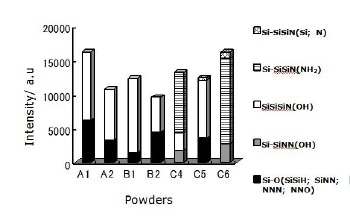
Figure 3. Components intensity of deconvoluted Si 2p signals for 0 sec etching time. The highest intensity fraction corresponds to configurations containing OH groups for five out of seven powders. The exceptions are the two powders that were washed (C4 and C6) corresponding to a configuration containing an NH2 group.
The largest intensity observed for four out of seven powders corresponds to Si-SiSiN(OH) configuration meanwhile for the powder produced by carbothermal reduction process using NH3 as nitriding media (B2) the intensity of this configuration is comparable to the group which includes one O atom as backbone; Si-O(SiNN; SiSiH; NNN; NNO) configurations and in the case of the two powders produced by nitridation of silicon with HF acid washing in the final treatment (C4 and C6) the main configuration is Si-SiSiN(NH2). For six powders OH groups were observed in Si-SiSiN(OH) tetrahedral, the exception is the powder produced by nitridation of silicon using N2 + H2 as nitriding media with HF acid washing in the final treatment (C6). In this powder the OH groups were present in tetrahedral containing more nitrogen; Si-SiNN(OH). Although the two powders produced by the same production process (nitridation of silicon) using the mixture of N2 + H2 as nitriding media (C5 & C6) show completely different configurations they have in common the presence of very low amounts of Si-SiSiN(Si; N) tetrahedral. The powder produced by diimide precipitation process using NH3(l) as nitriding media (A1) presents the highest intensity of Si-O(SiNN; SiSiH; NNN; NNO) tetrahedral and only the powder produced by nitridation of silicon using N2 + H2 as nitridation media with acid washing in the final treatment (C6) does not show this configuration. For the diimide precipitation process the use of NH3 gas (A2) instead of liquid (A1) as nitriding media results in a lower intensity of tetrahedral containing OH group (Si-SiSiN(OH)). The same is observed for carbothermal reduction process when NH3 gas (B2) is used instead of N2 (B1).
The intensity of each configuration in Si-O(SiNN; SiSiH; NNN; NNO) tetrahedral is shown in Figure 4. It is seen that for the powder produced by diimide precipitation using NH3(l) as nitriding media the largest intensity corresponds to Si-SiNNO. Tetrahedral configuration containing backbone hydrogen as Si-SiSiHO is observed only in the powder produced by nitridation of silicon using the mixture of N2 + H2 as nitriding media without washing (C5) and only the powder produced by carbothermal reduction process using N2 as nitriding media (B1) presents two O atoms backboned to Si.
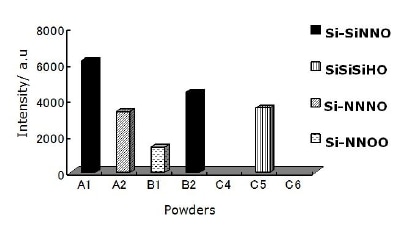
Figure 4. Components intensity of Si-O(NNN; SiSiH; SiNN; NNO) configurations for all as received powders. Only the two powders produced by nitridation of silicon with acid washing (C4 & C6) do not show O configurations.
For the C 1s signal, the powder produced by carbothermal reduction process using methane (CH4) for reduction (B2) shows the highest intensity, especially in C-H bond at 284.6 eV as seen in Figure 5, shown as an example of deconvoluted C 1s signal for this powder using the Gaussian curve fit. Three peaks were decomposed from measured XPS spectrum and identified. Two types of carbon bonds in addition to dominant C-H peak are located at 288.2 eV and 286.3 eV corresponding to C=O and C-O. C-Si and C-N bonds were not observed in this state. Three kinds of C bonds have been also observed in α-Si3N4 powders [2, 25]. Some of the oxygen signal, however, is associated with the background carbon signal from the adhesive tape used for supporting the powders and from residual contamination on the powders [25].
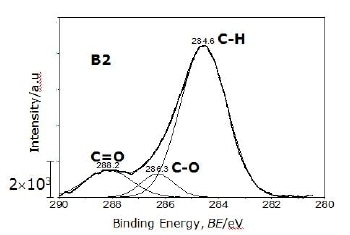
Figure 5. One example of deconvoluted C 1s signal (powder B2). Three kinds of C bonds were observed corresponding to C=O, C-O and C-H.
The assignment of all components in the Si 2p signal as described above agrees with the C 1s, N 1s and O 1s signals. The relations shown in Figures 6 and 7 may be taken as a confirmation of the tetrahedral assignments.
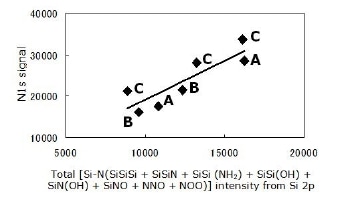
Figure 6. Agreement between two signals; Si 2p and N 1s, for all powders. All observed N atoms are bonded to Si. All configurations are described in this section
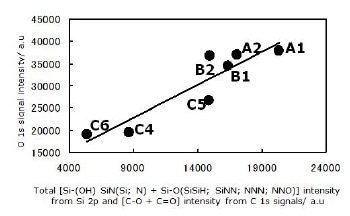
Figure 7. Agreement between three signals; Si 2p, C 1s and O 1s, for all powders. All observed O atoms are bonded to Si and C atoms. All configurations for Si atom are described in this section
Another representation of the data for the powders is shown in Figure 8. Configurations where OH, NH2 or O bond to silicon were grouped as follows: OH; SiSiN(Si;N)(OH), NH2; SiSiSiN(NH2) and O; SiO(NNN; SiSiH; SiNN; NNO). The Figure shows the fractions of each group for each powder obtained using the summation of intensities obtained from the deconvoluted spectra. For five out of the seven powders the largest configuration contains OH as Si-SiSiN(OH) and the second; O as SiO(NNN; SiSiH; SiNN; NNO) configurations. For the two powders that were washed (C4 & C6) the largest contains NH2 as Si-SiSiN(NH2) and the second; OH as SiSiN(Si;N)(OH) configurations respectively. The powder produced by carbothermal reduction process using NH3(g) as nitridation media shows the largest intensity fraction of O containing configurations, meanwhile the one using N2 (B1) shows the largest of OH configurations at the most outer surface.
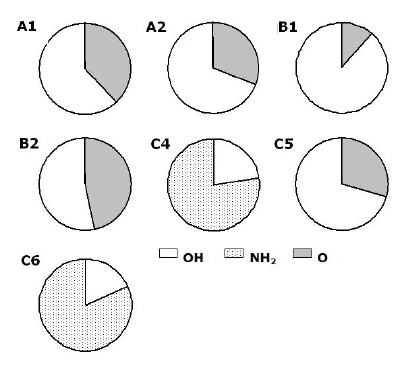
Figure 8. Main components at the most outer layer for all powders. The two main components present for all powders except for the two powders that were washed (C4 & C6) are O and OH containing configurations SiO(NNN; SiSiH; SiNN; NNO) and SiSiN(Si;N)(OH), respectively.
Summary and Conclusions
Tetrahedral structures Si-XYZW with different atoms or groups were observed for all as received commercial Si3N4 powders. For five out of the seven Si3N4 powders the two main tetrahedra present on the most outer surface layer are Si-SiSiN(OH) and tetrahedral containing O; Si-O(NNN; SiSiH; SiNN; NNO). The two powders produced by nitridation of silicon with HF acid washing in the final treatment (C4 and C6) present Si-SiSiN(NH2) and tetrahedral containing OH; Si-SiN(Si; N)(OH). The powder produced by carbothermal reduction process using NH3 (g) presents the highest fraction of O containing tetrahedra.. The results are in agreement with our previously reported [16] about the surface composition being a mixture of tetrahedral units Si-XYZW, XYZW representing Si, H, N and O atoms or OH, NH2 groups.
References
- 1. H. -J. Kleebe, H. Schmidt, W. Lehner and G. Ziegler, J. European Ceram. Soc., 22 (2002) 955 - 961.
- 2. M. N. Rahaman, Y. Boiteux and L. C. De Jonghe, Am. Chem. Soc. Bull., 65 [8] (1986) 1171 - 1176.
- 3. K. Okada, K. Fukuyama and Y. Kameshima, J. Am. Ceram. Soc., 78 [8] (1995) 2021 - 2026.
- 4. L. Bergstrom and R. J. Pugh, J. Am. Ceram. Soc., 72 [1] (1989) 103 - 109.
- 5. C. Morterra and G. Magnacca, Catalysis Today, 27 (1996) 497 - 532.
- 6. B. V. Zhmud, J. Sonnefeld and L. Bergstrom, Colloids and Surfaces, A158 (1999) 327 - 341.
- 7. G. Ramis, G. Busca, V. Lorenzelli, M. I. Baraton, T. Merle-Mejean and P. Quintard, In “Surfaces and Interfaces of Ceramic Materials" ed. L. C. Dufour, C. Monty and G. Petot-Ervas, Kluwer Academic, Dordrecht, the Netherlands, (1989) 173 - 184.
- 8. S. Prochazka and C. Greskovich, Ceramic Bulletin, 57 [6] (1978) 579 - 586.
- 9. P. Ho, Richard J. Buss and R. E. Loehman, J. Mater. Res., 4 [4] (1989) 873 - 881.
- 10. M. -I. Baraton, High Temp. Chem. Processes, 3 (1994) 545 - 554.
- 11. Y. L. Li, Y. Liang, F. Zheng, K. Xiao, Z. –Q. Hu, J. Mater. Sci. Lett., 14, (1995) 713 - 715.
- 12. E. A. Leone, S. Curran, M. E. Kotun, G. Carrasquillo, R. V. Weeren and S. C. Danforth, Communications of Am. Ceram. Soc., 79 [2] (1996) 513 - 517.
- 13. H. Schmidt, G. Nabert, G. Ziegler and H. Goretzki, J. Euro. Ceram. Soc., 15 (1995) 667 - 674.
- 14. T. Nakamatsu, Ch. Ishizaki and K. Ishizaki, Ceramic Engineering and Science Proceedings, published by American Ceramic Society, 19 [3] (1998) 3 - 10.
- 15. T. Nakamatsu, N. Saito, Ch. Ishizaki and K. Ishizaki, J. Euro. Ceram. Soc., 18 (1998) 1273 - 1279.
- 16. T. T. T. Hien, Ch. Ishizaki and K. Ishizaki, J. Ceram. Soc. Japan, 112 [1] (2004) 1 - 5.
- 17. J. Viard, E. Beche, D. Perarnau, R. Berjoan and J. Durand, J. European Ceram. Soc., 17 (1997) 2025 - 2028.
- 18. S. Miyazaki, Y. Hazama and M. Hirose, Optoelectronics-Devices and Technologies, 5 [1] (1990) 81 - 90.
- 19. J. Viard, E. Beche, J. Durand and Berjoan, J. Euro. Ceram. Soc., 17 (1997) 2029 - 2032.
- 20. J. Szepvolgyi, I. Mohai and J. Gubicza, J. Mater. Chem., 11 (2001) 859 - 863.
- 21. M. Peuckert and P. Greil, J. Mat. Sci., 22 (1987) 3717 - 3720.
- 22. J. R. Shallenberger, D. A. Cole and S. W. Novak, J. Vac. Sci. Technol. A, 17 [4] (1999) 1086 - 1090.
- 23. 23. I. V. Bertori, G. Mink, G. Szekely, T. Vaivads, J. Millers and T. Grabis, J. Surface & Interface Analysis, 12 [1-2] (1988) 527 - 530.
- 24. M. Takakura, T. Ogura, T. Hayashi and M. Hirose, Jpn. J. Appl. Phys., 27 [11] (1988) L2213 - L2215.
- 25. H. -W. Chen, D. Landheer, T. -S. Chao, J. E. Hulse and T. -Y. Huang, J. Electrochem. Soc., 148 [7] (2001) F140 - F147.
- 26. P. S. Wang, S. G. Malghan, S. M. Hsu and T. N. Wittberg, J. Mater. Res., 8 [12] (1993) 3168 - 3175.
Contact Details
Kozo Ishizaki
Nagaoka University of Technology (Nagaoka Gijutsu-Kagaku Daigaku) School of Mechanical Engineering, Nagaoka, Niigata 940-2188, Japan
E-mail: [email protected]
Tran Thi Thu Hien
Permanent address: Ha noi University of Technology (Dai Hoc Bach Khoa Ha noi), 1st Dai Co Viet Street, Hanoi, Vietnam
Chanel Ishizaki
Permanent address: Nano Tem Co. Ltd, Simogejo1 - 485, Nagaoka, Niigata 940 - 0012, Japan
This paper was also published in print form in “Advances in Technology of Materials and Materials Processing”, 10[1] (2009) 53-62.