Introduction Alumina (Al2O3) is one of the best ceramic structural materials. This non-metallic material has been widely studied in recent years [1, 2]. These studies have been prompted due to a large number of characteristic physical properties and also to different type of applications. These include properties such as: high wear resistance, resistance against high temperature corrosion, high electrical resistivity, high thermal conductivity and an already established ceramic-metal joining technology. This ceramic is already used as a structural sections subjected to mechanical loads usually at high temperatures. Alumina is also used as an effective electrical insulator. Different applications of this material usually requires particular associated properties design, therefore, studies to improve the mechanical strength, the electrical conductivity, the thermal diffusion, the resistance under corrosion environments etc., have been carried out in recent years. Most of these properties are strongly dependent on the processing route to obtain the thermodynamic stable α-alumina. The aluminum hydroxides (HA) and the oxyhydroxides (OHA) are the main resource materials to obtain the alumina. The most used names for these compounds are related with the mineralogical nomenclature [3]. Thus, for example, crystalline compounds such as Al2O3.xH2O where 1<x<2 are known as boehmite, however, when this compound has a poor crystallinity, the material is known as pseudoboehmite. The pseudoboehmite under heat similar to the transformations of the boehmite [4]. Few investigations have been carried out on the role of the pseudoboehmite as the structural induced phase of the α alumina. To get an insight on these structural heat induced transformation, a morphological and structural characterization of the heat induced intermediate phases is required. In this investigation the nature of these transformations are studied. Also, the shrinkage characteristics of the crystalline phases which are obtained under these transformations are explored. Experimental The pseudoboehmite was obtained using the U. G. process of the alunite [5]. This process is related with the alkaline desulphatation of the basic compounds [6]. Also, this compound can been obtained with the desulphatation of the Al2(SO4)3 using an ammonia solution. The obtained pseudoboehmite has a chemical composition of Al4O3(OH)6. Furthermore, the compound derived from the commercial sulphate with a chemical composition (wt%) given by: Al2O3 (98.9010), SiO2 (0.5560), CaO (0.4960), Fe2O3 (0.0259), ZnO (0.0061), CuO (0.0053), SO3 (0.0048), NiO (0.0032) and K2O (0.0015) [7]. The powders were annealed at 250, 550, 1000 and 1200°C. The crystalline phases were obtained using X-ray diffraction techniques (Siemens D5000). Morphological characteristics of the powders have been obtained using the scanning electron microscope SEM (Jeol 6400) also some microstructural characteristics were obtained with a transmission electron microscope TEM (Jeol 2010). TEM specimens were obtained from a suspension of the powders in isopropyl alcohol. Drops of this suspension were deposited on a copper grid (3 mm in diameter) with an amorphous carbon film. Dilatometer measurements (Dilatronic theta 6548) have also been used to determine the nature of the structural transformations of the pseudoboehmite. The specimens for the dilatometer measurements were small bars of 1cmX0.5cmX0.5cm. These bars were obtained using an axial pressing instrument. The velocity of the dilatometer experiments were carried out at 5oC/minute. Results and Discussion In order to obtain the structural transformation temperatures associated with this compound, dilatometer measurements have been carried out. Figure 1 shows the linear shrinkage (1a) and the corresponding derivative (1b) which represent the changes of the pseudoboehmite structure. The maximum transformation velocity of the pseudoboehmite to the γ-alumina is obtained at 500°C. The velocity of shrinkage is higher at 1200°C. This corresponds to the θ to α-alumina transformation. The initial features of the sintering process can be observed at 1500°C. These experimental dilatometer results were used for the setting of the annealed temperatures of the pseudoboehmite powders. Figure 2 shows a bright field image and its corresponding electron diffraction pattern. The image contrast shows the characteristic spike morphology of the pseudoboehmite. Figure 3 shows the X-ray diffraction patterns obtained from the pseudoboehmite and also from the annealed specimens at 550°C, 1000°C and 1200°C (c, b and a) respectively. Figure 3d shows the typical spectra of the boehmite. However, the peaks are appreciably wide in shape. This effect is related with the poor crystallization of this phase. This is the main reason for the name pseudoboehmite. Figure 3c shows the spectra which correspond to the γ-alumina obtained at 550°C. Furthermore, Figure 3b shows the θ- alumina obtained at 1000°C. 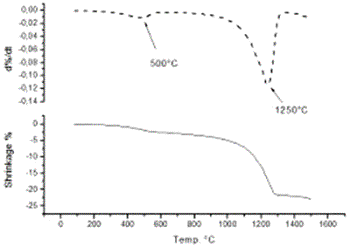 | Figure 1. Linear shrinkage of the pseudoboehmite sample (1a) and its corresponding derivative (1b). Structural transformations are obtained at 500°C and also at 1250°C. | 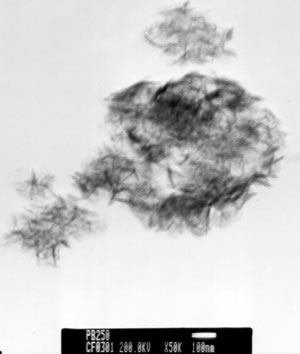 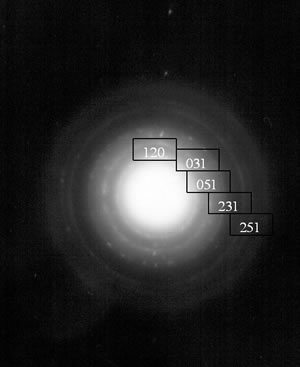 | Figure 2. Bright field image and its corresponding electron diffraction pattern of the pseudoboehmite. The characteristic spike morphology of the pseudoboehmite can clearly be seen. | 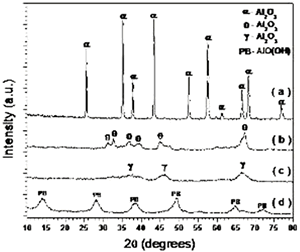 | Figure 3. X-ray diffraction pattern of the α-phase (3a), the θ-phase (3b), the γ-phase (3c) and the pseudoboehmite (3d). The θ, γ and pseudoboehmite phases present poor crystallinity properties. However, the α-phase spectrum has clear indications of a enhanced crystallinity. | 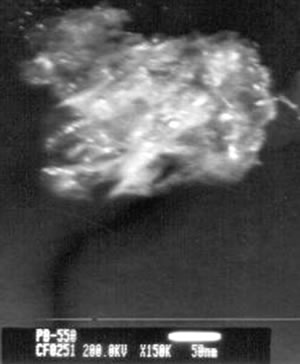 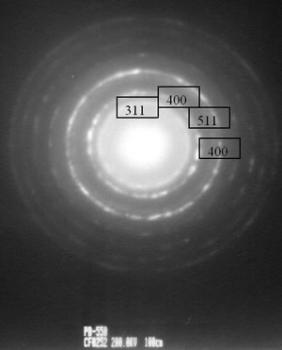 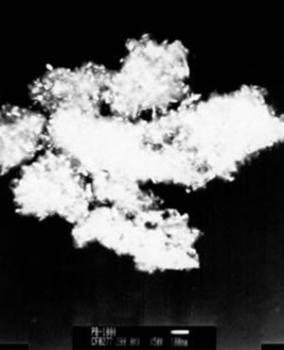 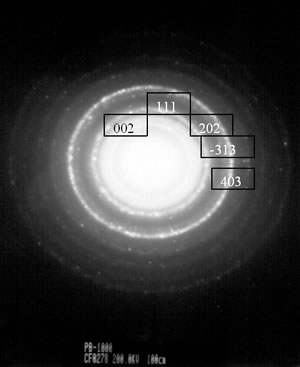 | Figure 4. TEM images of the γ and θ phases and their corresponding electron diffraction patterns. The images illustrate the nanometric nature of the alumina crystals and also their whisker morphology. | Both of these phases (γ and θ) still show appreciably wide peaks which indicates poor crystalline conditions. It’s important to note that the crystalline state of the γ and θ phases is lowest in comparision with the pseudoboehmite phase. However, Figure 3a shows the spectra which corresponds to the α-alumina. This spectrum was obtained from the pseudoboehmite powders annealed at 1200°C. The peaks in the spectra are narrow in shape, suggesting good crystalline conditions. Some reflections in the γ-alumina spectra are also obtained in the θ- alumina spectra. This result is related with the structural transformation of the cubic structure (γ-phase) to the monoclinic (θ-phase) structure. The crystalline nature of the different phases is strongly dependent on the annealed process. It is important to point out that both, the dilatometer experimental results and the X ray diffraction patterns do not show any evidence of the δ alumina presence. The Table 1 shows the X-ray crystallographic values and also those obtained after a minimum square refinement of the experimentally measured values. The lattice parameters show a slight increment in their values, consequently there is also an increment in the unit cell volumes. These variations could be related to impurities obtained during the processing route. TEM observations of the annealed powders of the pseudoboehmite have also been carried out. Figure 4 shows the TEM images and also the diffraction patterns which corresponds to the γ and θ alumina phases. These images illustrate the nanometric nature of the alumina precursors and also the whiskers characteristic shapes. Both, the XRD results and the TEM observations show the presence of nanometric crystals in the pseudoboehmite phase. X ray diffraction results and TEM observations obtained from the γ and the θ phases suggest an apparent dependence on the structural characteristics of the pseudoboehmite. Figure 5 shows the bright and dark field images of an alumina crystal. Also, its corresponding diffraction pattern is illustrated. In contrast to Figure 4, these α-alumina images shows larger crystalline grains with sizes of a few hundred nanometers. 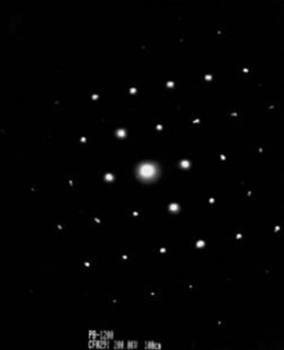 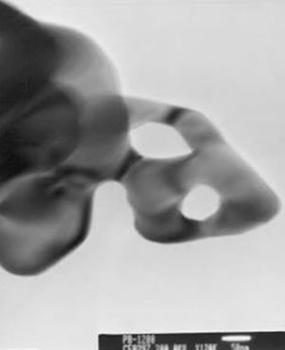 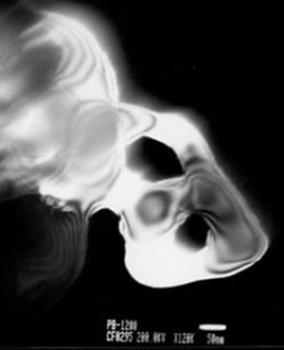 | Figure 5. Bright and dark field image of an α- alumina crystal. Its diffraction pattern is also illustrated. The crystal size is higher than 500 nanometers. | This result seems to be in agreement with the X-ray spectra corresponding to this crystalline phase (Figure 3a). Some annealed specimens show the presence of different crystalline structures. This is illustrated in Figure 6. This figure shows a dark field image and its corresponding diffraction pattern from a specimen annealed at 550°C. 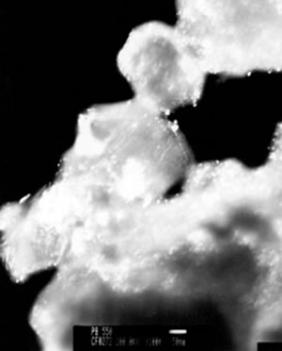 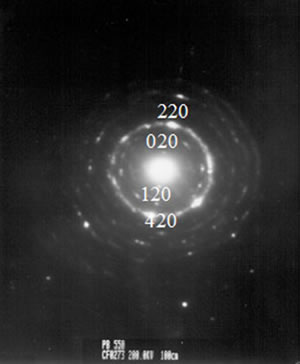 | Figure 6. Dark field image of a pseudoboehmite specimen annealed at 550°C. The diffraction pattern suggests the presence of pseudoboehmite and also γ-alumina. | 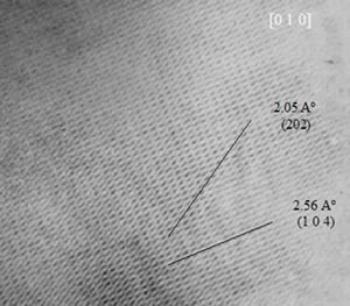 | Figure 7. HREM image of an α-alumina specimen. The HREM image shows interplanar distances which correspond to this crystalline structure (α-alumina). | The indexation of the diffraction pattern suggests the presence of pseudoboehmite and also γ-alumina. The presence of α-alumina has also been obtained from the high resolution electron microscope (HREM) images. This is illustrated in Figure 7 which correspond to the specimen annealed at 1200°C. The HREM image shows interplanar distances from the α-alumina crystalline specimen. The XRD results and the TEM observations suggest a high crystallinity nature of the α-alumina phase. The precursor phases of the α-alumina have nanometric crystalline structures. Therefore, these phases have a strong influence on the structural nature of the α-alumina phase. The heat treatments or sintering of the precursors powders have suggested the processing routes to obtain α-alumina with nanometric crystalline sizes, which will improve the mechanical properties of this material. The absence of the δ phase and the less energy used to produce nanometric crystals of α-alumina, could have also some commercial implications along the production costs of α-alumina. Table 1. Lattice parameters variations of the different phases in comparison with the values from the X-ray crystallographic tables. | Phase | a | b | c | Vc | a | B | c | Vc | α-hexagonal | 4.7592 | 4.7592 | 12.9920 | 254.84 | 4.7643 | 4.7643 | 13.0083 | 255.71 | θ-monoclinic | 11.813 | 2.9060 | 5.6250 | 187.281 | 11.8267 | 2.9042 | 5.6380 | 187.816 | Conclusions The microstructural characteristics of the polymorphic phases during the transformation and the crystallization of the pseudoboehmite to the α-alumina phase (P-γ-θ-α-Al2O3) have been studied. The α alumina precursor phases (pseudoboehmite, γ phase and θ phase) have crystal sizes in the nanometric range. The nanometric crystalline sizes of the precursors phases suggest the control of microstructural evolution during the formation of α-alumina phase. Also, an increment in the lattice parameters of the alumina phases has been obtained. This increment could be related with the presence of impurities which have been produced during the synthesized process. Acknowledgements G. Rosas thank the UMSNH and CONACYT (38410-U) for financial support. References 1. D. Lee William and W. Mark Rainforth, “Ceramic microstructures. property control by processing”, Chapman & Hall, (1994) 3. 2. J. Alexander Chediak, “Ceramic Engineers in the 21st Century”, The American Ceramic Society Bulletin, 75 [1] (1996). 3. R Tertian, and D. Papee, “Thermal & Hidrothermal Transformations of Alumina”, J. Phys. Chem., 55 (1958) 341-353. 4. K. Wefers and C. Misra, “Alcoa Tech Reporte # 9”, Alcoa Tech Center, PA, USA (1987). 5. López W. X., “Three methods to produce alumina from alunite”, Light Metals., 2 (1977) 49-58. 6. H. Juárez-M, J. M. Martínez-R. and J. M. Ruvalcaba-L., “Obtención y Caracterización de Pseudoboehmita a Partir de Sulfato de Aluminio Comercial", IV Congreso Iberoamericano de Química Inorgánica y XI Congreso Mexicano de Química Inorgánica, Guanajuato, Gto., México, (1993) 256-260. 7. S. D. De la Torre, A. Kakitsuji, H. Miyamoto, K. Miyamoto, H. Balmori-R., J. Zárate-M. and M. E. Contreras, “Seeding with α-Alumina for Transformation and Densification of Boehmite-Derived γ and δ-Alumina by Spark Plasma Sintering”, Ceramic Transactions, 94 (1999) 83-89. Contact Details |