Continuous exploration of advanced magnetic and semiconductor materials is essential to meet the increasing demand for higher performance electronics with lower power consumption and better bandwidth.
Characterizing a material’s transport properties is the starting point for such research works. I-V and C-V techniques are initially used for evaluation of fundamental electrical properties, including conductivity and impedance. Following this, more sophisticated analyses like Hall effect measurement are performed for determination of transport properties such as carrier type, mobility and concentration.
The use of X-ray, Raman, UV and THz spectroscopic techniques allows more details to be gained about the properties of a material. Due to the limited value of simple bench-top measurements when performed at room temperature, it is necessary to measure the material response under a wide range of temperatures to obtain the greatest insight from these early-stage characterization techniques.
The ability of altering the magnetic field observed by the sample is also required to characterize a material with multiple carrier types or explore magneto-transport properties.
Cryogenics and Material Characterization
Key insights into basic conductivity mechanisms can be obtained when a new semiconductor material is characterized across a range of temperatures. Thermally activated carriers in a material become immobilized at the lowest cryogenic temperatures, thereby enabling to reveal the material’s inherent electronic transport properties.
The performance of a device will be negatively affected when carriers are trapped by defects in the lattice structure of the material. Researchers can observe the transport characteristics of a material at cryogenic temperatures to gain insights into trapped charge concentration, movement and location.
The effect of temperature on conduction properties is more pronounced in superconducting materials. Magnetization shifts can be observed in some magnetic materials, including ferromagnetic nanoparticles, due to temperature effect.
The Versatile Cryogenic Probe Station
Different cryogenic research platforms are available to perform variable temperature study. Some of them offer integrated magnets. The cryogenic probe station shown in Figure 1 is the most versatile system, providing the required variable temperature and magnetic field control in an easily accessible environmental chamber. Individual diced samples up to full wafers can be supported by the chamber.
.png)
Figure 1. A cryogenic probe station (Lake Shore Cryotronics Model CRX- VF).
Based on the requirement, it is possible to land and reposition the micro-manipulated probes on the sample in the chamber in a non-destructive manner, thus enabling unprecedented measurement flexibility (Figure 2). The elimination of cumbersome wire bonding to smaller diced samples, in turn, eliminates any potential sample damage.
.jpg)
Figure 2. Three-terminal DC probing configuration for transistor measurements.
In cryogenic probe stations, researchers have to perform analyses manually by coupling their desired equipment to the probe arms. In recent times, some key probe station suppliers have started providing optional measurement packages, which enable performing highly complex characterization like the Hall effect measurement.
These packages have a probe station’s inherent flexibility to facilitate a wide range of measurements and a turn-key system’s time-saving advantages by automating certain measurement protocols and integrating analysis tools for accurate determination of desired material parameters.
Non-Destructive Wafer-Scale Probing
Whether determining basic material properties transitions or early stage device development, it is ideal to use the cryogenic probe station for device performance characterization. Considering the requirement for a high level of lab effort to build device structures, characterization process is becoming increasingly important in order to maintain wafer integrity. Mid-process checks of properties such as mobility and conductivity are also useful in the development of devices.
However, these wafer-scale evaluations can be conveniently performed, thanks to the cryogenic probe station’s non- destructive probing capability. For Hall structure measurement, the size of test structure could vary from tens of microns up to tens of millimeters.
Moreover, active material surfaces and delicate organic materials are protected from contamination caused by moisture or atmosphere owing to the sample chamber’s vacuum environment. The probe station platform’s ability to integrate load-lock structures and even directly with deposition systems makes the system more versatile.
The combination of vertical magnetic field probe stations and sophisticated mobility analysis software tools is useful to analyze multilayer or multi-carrier semiconductor Hall samples through segregation of the mobility spectrum for every carrier species. The identification of individual carriers allows users to correct for unwanted contaminants during growth, corroborate doping effectiveness, and perform quality control during different production stages.
Example: Cryogenic Probing of pHEMTs
The complex interactions between the device geometry and material properties make pseudomorphic high-electron mobility transistor (pHEMT) devices vulnerable to off-state breakdowns, limiting the device’s maximum power handling capability.
Different physical mechanisms have been proposed for the breakdown with unique temperature dependencies, thus enabling to differentiate the mechanism dominating in a specific device architecture. GaAs pHEMTs employed in RF applications are subjected to variable temperature, on-wafer transport measurements.
Figure 3 shows three distinct gate leakage regimes observed in the temperature-dependent gate current for fixed source-gate voltages. The gate current becomes nonmonotonic temperature dependence above 210K, indicating a thermionic field emission mechanism.
The gate current becomes source gate voltage dependence at temperatures less than 150K, but is temperature independent, suggesting the domination of tunneling transport on the barrier conduction. The temperature dependence of the gate current observed between 150 and 210K is indicative of an intermediate breakdown mechanism, most likely defect-assisted tunneling.
.png)
Figure 3. Temperature-dependent pHEMT gate current during measurement of transfer curves (VDS = 3 V).
Continuous Wave Terahertz
Terahertz or THz, normally in the range of 300–3000GHz is the least explored electromagnetic spectrum. Since terahertz-frequency energy levels are in line with most magnetic and semiconductor materials’ inherent resonant characteristics, it is possible to stimulate and reveal the conduction and spin characteristics with more finesse.
Terahertz-frequency spectroscopy is gaining attention as a technique of choice for characterization of exotic material properties. This non-destructive, quasi-optical method is suitable to explore the conductivity of certain ultrathin epilayers, bulk semiconductors, and buried thin films in pre-device stage heterostructures.
THz spectrometers are available in both conventional time-domain (TDS) and next-gen frequency-domain (continuous wave or CW) versions. The advanced CW systems are advantageous in terms of resolution and cost over TDS, with the added advantage of allowing material analysis over a narrow frequency band of interest or at a single “dwell” frequency.
The requirements of physical and chemical materials analysis can be met with room-temperature THz spectroscopy. However, variable (cryogenic) temperatures and fields are required for the analysis of magnetics and electronics.
Signal power is often the challenge that needs to be taken care of in the THz spectroscopy. As a result, aligning source and detector photomixers becomes crucial and introducing multiple intermediate surfaces is unfeasible. The development of special cryogenically stable miniature CW-THz photomixers can address this problem by allowing direct introduction into high-field cryostats, as shown in Figure 4.
.jpg)
Figure 4. Continuous wave-THz spectroscopy system emitter and detector devices for semiconductor materials characterization
The repeated sample movement in and out of the THz beam as background reference is taken care of by automated sample stages, enabling logging of sample transmission measurements at each desired temperature and field step. Besides the amplitude spectra, the sample’s corresponding phase response is obtained and charted. This associated phase information is important to determine transport characteristics and other properties.
CW-THz Magneto-Spectroscopy
Several spectroscopic techniques are being used to explore new growth methods and materials, such as UV-vis to gain insights into band gaps and X-ray to explore crystalline structure. Various features of a new material, such as the interactions between structure, magnetism and charge in complex oxide systems, or a semiconductor’s frequency-dependent complex conductivity, can be explored with THz characterization.
From the transmission spectra acquired, numerically extracting complex-valued refractive index of a semiconductor is the first step in the determination of its conductivity. The application of the Drude model fit to the refractive index allows evaluating the carrier concentration, average carrier scattering time, and mobility for most materials.
Cyclotron resonance (CR) of high mobility semiconductors can be mapped by measuring their THz response as a function of magnetic field. Band mass and carrier type can then be accurately determined from the CR’s linewidth and resonant frequency.
THz magneto-spectroscopy can help determining the mobility and carrier type even in lower mobility semiconductors consisting of larger band mass carriers, where CR may be not within the THz range. The magnetic field’s strength and direction influence the characteristics of electrons and holes for certain polarizations of the CW-THz wave (Figure 5). Algorithms are being developed by researchers for extracting mobility from field dependent CW-THz measurements.
.png)
Figure 5. Field-dependent THz spectra of a B-doped silicon substrate
Conclusion
The role of electronic and magnetic technology research is highly significant in the fabrication of high-performance computing and communications components. Non-destructive characterization systems equipped with variable cryogenic temperature and magnetic field environments are ideal to explore new magnetic and electronic materials.
CW-THz characterization systems are capable of aligning with the inherent resonances of various important physical phenomena, making them a potential tool to explore new characteristics of novel materials.
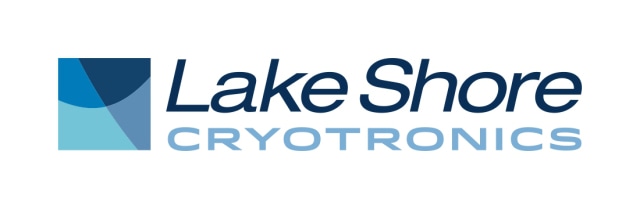
This information has been sourced, reviewed and adapted from materials provided by Lake Shore Cryotronics Inc.
For more information on this source, please visit Lake Shore Cryotronics Inc