XPS is an enabling technology which yields empirically quantitative information regarding the composition of compound semiconductor alloys. A study using Kratos AXIS Nova spectrometer to analyze novel SPLED structures to characterize Al content was undertaken. A depth profile for a full device was acquired using conventional depth profiling methods with Shard stepwise rotation. The Al content confirmed the expected compositions for the different layers and will be useful for modeling the performance of cell structures.
Compound semiconductors are the main underpinning technology in optoelectronics. They are used in electronic applications with specialist requirements such as power. The ability to engineer the electronic and optical properties of compound semiconductor alloys, regarding their alloy composition, which may be binary, ternary, quaternary or quinary, and heterostructures, is a key part of their success. According to alliedmarketresearch.com, the global compound semiconductor market was worth $66bn in 2016 and predicted to be worth $143bn by 2023. However, despite the clearly important role of composition in compound semiconductors, its accurate determination remains a challenge. This is especially true for devices where there are many different layers.
Extreme examples of this are devices that contain distributed Bragg reflectors: alternating layers of high- and low-refractive-index material to create a stop-band in which a set of wavelengths are almost fully reflected. For example, vertical cavity surface emitting lasers (VCSELs) are inexpensive semiconductor lasers that use a pair of DBRs to form the mirrors of the lasing cavity.
Demand for VCSEL application of communications continues to rise, while the non-communication market is expected to increase nearly 8-fold by 2022. The quality and consistency of the DBRs are known to be important. This is because a VCSEL has a gain length on average 105 times smaller than an edge-emitting laser, and therefore needs ultra-high reflectivity mirrors to achieve a reasonable threshold current. Other emerging devices that use DBRs are single photon LEDs (SPLEDs) which are needed for quantum key distribution in quantum cryptography networks.
This study focuses on these DBRs, and methods to accurately characterize their structure. This includes determining whether the semiconductor layer growth has proceeded as anticipated. To further characterize the growth, X-ray photoelectron spectroscopy (XPS) depth profiles are taken to measure the chemical composition of the DBR layers. Even a small change in Al composition affects the refractive index, which, in turn, changes the optical path length of the layer. Furthermore, this has consequences for everything from mirror characteristics to laser output wavelength. The device performance is directly related to XPS yields quantitative information regarding Al content for the DBR structure.
Experimental
XPS analysis was performed using the Kratos AXIS Nova spectrometer on the SPLED structure that was grown by molecular beam epitaxy at Lancaster University, UK. The Minibeam IV focused ion gun was used for depth profiling in 4 keV Ar+ mode. The ion source has a bend in the ion column to remove energetic neutrals to prevent the degradation of the interface resolution of this multilayer sample. To minimize sample roughening during the etching, a sample rotation using 90˚ increments at each etch cycle was used. Compucentric rotation about a point during etching can be implemented by a simple tick-box in the ESCApe acquisition software.
.jpg)
Figure 1A. Complete depth profile of SPLED structure.
.jpg)
Figure 1B. Zoomed-in emitter region.
Results
The SPLED wafer was mounted onto the rotation platen before it was loaded into the instrument. Depth profiling was performed using high-energy monatomic 4 keV Ar+ ions with the focused beam rastered across the surface to form a uniform etch crater. To determine the composition of the newly exposed surface, XP spectra were acquired after each etch cycle. Small-spot spectroscopy was utilized to limit the influence of crater edge effects on the interface resolution.
As a result, the alternating GaAs/Alx Ga1-x As layers can be seen by the dramatic variation in Al content. In this particular structure the Alx Ga1-x As layers were designed to have an Al fractional content, x, of 0.9 and an elemental content of 45%. The XPS quantification showed a consistent value of ~46% for the Alx Ga1-x As layers which is well within the accuracy of the method.
Importantly, the composition of the emitter layer is consistent with the desired composition, although, after etching through the material for several further mirror layers the profile begins to lose form. This is most likely due to blending and roughening of layers due to the ion etching process itself. This is a known issue for this mode of etching. Unfortunately, due to the total thickness of the layers it was not feasible to repeat the experiment with low energy ion (250-500eV). This was due to the significant increase that it would cause in experiment time. Therefore, post-acquisition XP images were acquired for the Al 2p and Ga 3d photoelectron lines to determine the etch crater shape and positioning. The images clearly showed the layer structure of the device. In addition to this the images show the presence of the emitter layer 8 repeat units into the device.
.jpg)
Figure 2. Stitched XPS image of etch crater; Ga (RED) Al (BLUE).
Acknowledgments
Many thanks go to Tom Wilson, Peter Hodgson, Alex Robson and Manus Hayne for sample preparation and fruitful discussions, who would like to acknowledge EPSRC (grant number EP/P034233/1) and to IQE plc for financial support, through a CASE Award for Tom Wilson.
References and Further Reading
- alliedmarketresearch.com/compound-smiconductor-market, accessed 2/2/2018.
- yole.fr/IR_LED_LASER_MARKET_TRENDS.aspx#.WnSRQklLFaQ, accessed 2/2/2018.
- P. Moser, “Energy efficient oxide confined VCSELs for optical interconnects in data centers and supercomputers”, PhD thesis, Technische Universitt Berlin, Fakultt II - Mathematik und Naturwissenschaften (2015).
- paneuropeannetworks.com/science-technology/quantum-rings-torule-them-all/, accessed 2/2/2018.
- lancaster.ac.uk/physics/research/experimental-condensed-matter/ quantum-nanotechnology/, accessed 2/2/2018.
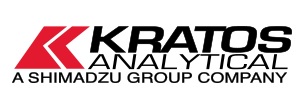
This information has been sourced, reviewed and adapted from materials provided by Kratos Analytical, Ltd.
For more information on this source, please visit Kratos Analytical, Ltd.