Fluorescence spectroscopy is a powerful tool that is used to identify the presence of both inorganic and organic molecules in complex systems. This can be achieved in one of two ways: either utilizing a process known as autofluorescence, which uses the fluorescence properties inherent to the molecule, or by introducing a molecular tag into the system, which has an affinity for the molecule of interest and a known fluorescence spectrum.
The fluorescent tags are known as fluorophores and can be engineered with specific emission and absorption bands. This means that multiplexing can be used to detect a broad range of species in a single analyte. This technique is used widely in many biomedical and biological applications which include sequencing of DNA.
When used together with confocal microscopy, this technique produces multispectral images of small objects including cells. Fluorescence spectroscopy is also applied in the field of anti-counterfeiting. Here, fluorescent tags are added to the ink of the currency and will emit a unique fluorescence spectrum, known only to the manufacturer, when illuminated by the proper excitation wavelength.
.jpg)
Theory of Fluorescence Spectroscopy
It is useful to briefly explore the fundamental physics involved in fluorescence spectroscopy in order to understand its full power. A molecule has to absorb a photon with enough energy to excite the system from the ground state (S0) to the next electronic energy level (S1) before the fluorescence process can occur.
When the molecule is in the excited state it releases the excess energy as quickly as possible in order for it to relax back down to the ground state which is more stable. The excess energy is converted into a mixture of photons and phonons and this is called radioactive or non-radioactive decay.
Figure 1 shows that, in the case of fluorescence emission, the molecule will initially drop to a lower vibrational state non-radiatively in the S1 band and then decay radioactively to the S0 band. The emitted photon from this process has less energy than the absorbed photon so it ‘shifts’ to a longer wavelength than the absorbed photon. This wavelength shift is known as Stokes shift and is shown in the Jablonski diagram of electronic states.
.jpg)
Figure 1. Example Jablonski diagram and the fluoresces absorption/emission spectra of a typical fluorescent molecule.
The energy differential between S0 and S1 and the intraband vibrational mode structure depend highly on the structure of the molecular bond. This dependence is particularly high in the presence of double or triple bonds as these exploit loosely bound p-electrons. Consequently, complex organic and biological molecules often exhibit high autofluorescence. As a result of this, the life sciences were among the earliest to adapt fluorescence spectroscopy and this enables the design of fluorophores, large organic molecules with specific absorption and emission properties, that are tailored for specific applications.
These fluorophores can be designed to bond with molecules of specific interest and so create fluorescence tags that are chemically sensitive, such as those previously mentioned for sequencing DNA. Recently, quantum dots have become popular in fluorescence applications. Here, the emission spectra are dependent on the size of the quantum dot itself, rather than the molecular structure of the material. Over the years, the cost and complexity of nanofabrication have decreased and so quantum dots are increasingly being used for a wide variety of applications in fluorescence, for example in anti-counterfeiting.
Typical Measurement Configurations
Fluorescence spectroscopy can be used in a broad range of applications and so the instrumental configurations also vary greatly between applications. As a result, most researchers favour configurable components which can be swapped easily in and out, depending on the requirements of the application specified. Avantes has worked closely with many process engineers and researchers in order to facilitate fluorescence measurement in clinical, laboratory, and industrial environments using fiber coupled miniature spectrometers, accessories and light sources.
Avantes has developed a full suite of fluorescence spectroscopy configurations over this period which have been used by researchers in both translational and laboratory research. Lots of these configurations have also been the basis for a broad variety of commercial products which use Avantes’ OEM spectrometers as their measuring instrument.
Relatively low-energy levels are associated with fluorescence spectroscopy compared to other methods such as UV/Vis spectroscopy. Therefore it is generally preferable to choose a high sensitivity spectrometer, for example the AvaSpec-HS2048XL-EVO or the AvaSpec-Hero. Higher sensitivity spectrometers are of particular use in more demanding applications, for example the detection of low concentration fluorophores in a sample matrix or the measurement of autofluorescence in tissues.
When compared to other low-light spectroscopy, for example diffuse reflection spectroscopy and Raman spectroscopy, fluorescence has relatively broad emission bands. These allow for spectroscopists working with samples that are strongly fluorescent to consider using a less sensitive spectrometer, for example the AvaSpec-Mini2048CL or the AvaSpec-ULS2048CL-EVO, which have a wide entrance slit to allow more light into the spectrometer.
Most spectrometers from Avantes come with an option for replaceable entrance slits and this allows the user to determine the optimal entrance slit width experimentally for each specific fluorescence application. This further enhances the flexibility that fiber coupled miniature spectrometers provides.
Cuvette-Based Measurements
The simplest fluorescence spectroscopy set-up uses a fiber coupled cuvette holder with orthogonal connection ports so in order to collect spectra from a sample in a solution in a cuvette that is the standard 10 ml. Most small molecule solvents, such as alcohol, cyclohexane and water have virtually no autofluorescence signal. This means that the sample and the fluorophore can be dissolved in the solution for ease of handling.
Although similar to typical UV/Vis measurements, in a configuration for fluorescence measurement, the collection port and the excitation port must be orthogonal (at 90 degree angles) to each other, shown in Figure 2. This is to prevent the fluorescence signal being overpowered by light from the excitation source. The port in line with the excitation source must be left open when using this configuration for fluorescence measurement in order to prevent the transmitted light from reflecting around inside the cuvette holder.
It may also be connected to a second spectrometer so as to measure the UV/Vis absorption spectrum at the same time, or capped with a mirror to create a double pass cell. When performing quality control during the manufacture of fluorescent dyes and quantum dots, the dual UV/Vis and fluorescence capability of this simple component set-up is particularly useful. This is because it enables the excitation and emission properties of the molecule to be determined simultaneously.
.jpg)
Figure 2. CUV-UV/VIS-TC-Temperature controlled cuvette cell holder from Avantes.
Furthermore, a thermoelectrically controlled cuvette holder, as shown in Figure 2, gives the operator precise control over the temperature of the sample between -30 oC and +105 oC at an accuracy of 0.05 oC. As well as the ability to stabilize the temperature of the sample during measurement, this type of cuvette holder allows for the sample temperature to be varied in real time. This means that the effects of temperature on the emission spectra can be quantified.
Probe-Based Measurements
Although cuvettes work well for many applications, removing a sample for testing purposes is not always desirable. One alternative, when testing powder or liquid samples, is to use a fiber optic fluorescence probe, like the one illustrated in Figure 3. The probes use a bifurcated design and this allows the spectrometer to be coupled to one leg and the excitation source to the other leg.
These probes can have many different configurations of the internal fibers, such as in Figure 3 where twelve 200 micron core fibers surrounding one 600 micron core fiber. They all have one thing in common however, and that is that the collection and excitation paths at the sample are both colinear. This simplifies the probe design dramatically, allows for a compact form factor, and does not cause increased excitation signal collection as in the orthogonal approach used by a cuvette holder.
.jpg)
Figure 3. FCR-UV200/600-2-IND – Fluorescence probe from Avantes.
It becomes necessary to add additional filtration steps along the collection path in order to mitigate the collection issues associated with this configuration of Fluorescence system. Avantes offers both incline and direct attached filter holders and these allow the user to filter the signal on both the collection and excitation paths. Placing a bandpass filter in the excitation line and only allowing light in the excitation band into the probe will typically accomplish this.
However, since there is no such thing as a perfect filter, it is often necessary to add a long pass filter on the collection path in order to further reduce the excess light from the excitation source. An example set-up, where the FH-DA direct attach filter holder is attached to the excitation light source, and an FH-INL inline filter holder is inserted between the collection leg of the bifurcated fiber and a patch cord connected to the spectrometer, is shown in Figure 4.
.jpg)
Figure 4. Typical set-up fluorescence probe configuration.
As well as performing standard immersion measurements, fluorescence probes are also ideal for in situ testing in a broad range of fields from archaeological research to biomedical diagnostics. The probe fiber configuration for each of these applications may differ slightly from the example in Figure 2, but the overall principle is consistent. Fluorescence probes can be used uniquely in dental curing, where the ultraviolet light source serves as both the catalyst for curing and as the excitation source for the fluorescence measurement.
These probes can also be utilized in art authentication, where insight into pigments used in the production of a painting can be provided by the fluorescence spectra. This allows investigators to work out whether the pain used matches the period in which the art was allegedly produced. However, overexposure to ultraviolet light can cause damage to the pigments themselves and so additional care is required when ensuring the integrity of the work.
Fluorescence Microscopy
Fluorescence microscopy dates back to the early 20th century and coincides with the invention of the ultraviolet microscope, in 1911. Early researchers I the field quickly identified that the ultraviolet excitation, which had been originally chosen to try and increase spatial resolution of the images, was inducing autofluorescence in their samples. By 1914 it had been demonstrated that fluorophores could be created to bind living cells and this allowed molecular information to be gathered on the microscopic level for the first time.
Nowadays, fluorescence microscopy has become one of the most broadly used techniques in biological sciences and provides high-resolution hyperspectral imaging of living cells and other biological samples. Although in most cases, the excitation source is a single mode laser which allows for diffraction limited imaging, the spectrometer requirements are otherwise basically identical to the other configuration discussed earlier. Avantes offers three standard microscope adaptors and these allow the user to connect a miniature spectrometer easily to any standard microscopy post using a fiber optic patch cord.
Final Thoughts
Avantes offers a broad variety of spectrometers and accessories that are ideal for both OEM and laboratory fluorescence spectroscopy applications including the AvaSpec-HSC1024X58-TEC (AvaSpec-Hero), AvaSpec-ULS2048XL-EVO, and AvaSpec-HS2048XL-EVO. Each of the spectrometers is available as an OEM module, a stand-alone unit, or can be integrated into a multichannel rack mount system that combines excitation source and spectrometer(s) in a single housing – making them ideal for inline systems or custom-designed laboratories.
The AvaSpec instrument line communicates via Ethernet, RS-232, USB3.0 and allows active digital and analog input/output capabilities, providing a multitude of interface options with other devices. Furthermore, users can develop their own code for fluorescence applications using the AvaSpec DLL software development package, with sample programs in MatLab, Labview, C#, C++, Visual Basic, Delphi, and many other programming environments.
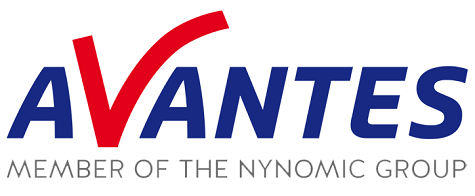
This information has been sourced, reviewed and adapted from materials provided by Avantes BV.
For more information on this source, please visit Avantes BV.