In this interview, Lena Woodis of Thermo Fisher Scientific discusses solutions by which to accelerate battery research and development.
Can you start by discussing the challenges of current battery technology?
Addressing the demand for improved performance and reduced costs in battery technology poses significant challenges. The Department of Energy has outlined many of the research goals aimed at discovering solutions to overcome these challenges.
Many of these objectives center around new materials for battery technology, new chemistries that enable wider applications of battery technology, and applied research aimed at enhancing diagnostics, battery testing, and lifetime testing for currently available commercial batteries.
What is vibrational spectroscopy?
Vibrational spectroscopy is an incredibly powerful analytical technique for materials analysis, providing the structure-function connection between material structure and functionality.
Fundamental questions about material identification can be answered quickly and easily by quantifying how much of the materials are present, and in what form they are present. This can be achieved with minimal to often no sample preparation.
What is the benefit of vibrational spectroscopy in battery research?
Vibrational spectroscopy enables very rapid screening of new chemistries and materials. This technique can be applied to a wide range of sample forms—just about anything you can imagine can be probed with vibrational spectroscopy.
There is a wealth of information in a vibrational spectrum. The intensity of the collected peaks provides information about the quantity of the material present. The identification of materials and determination of their molecular structure are derived from the position and presence of the peaks present.
By comparing spectra, analyzing peak shifts, and observing relative peak widths, you can obtain information about the three-dimensional structure of the material and understand how the physical and chemical properties of that material related.
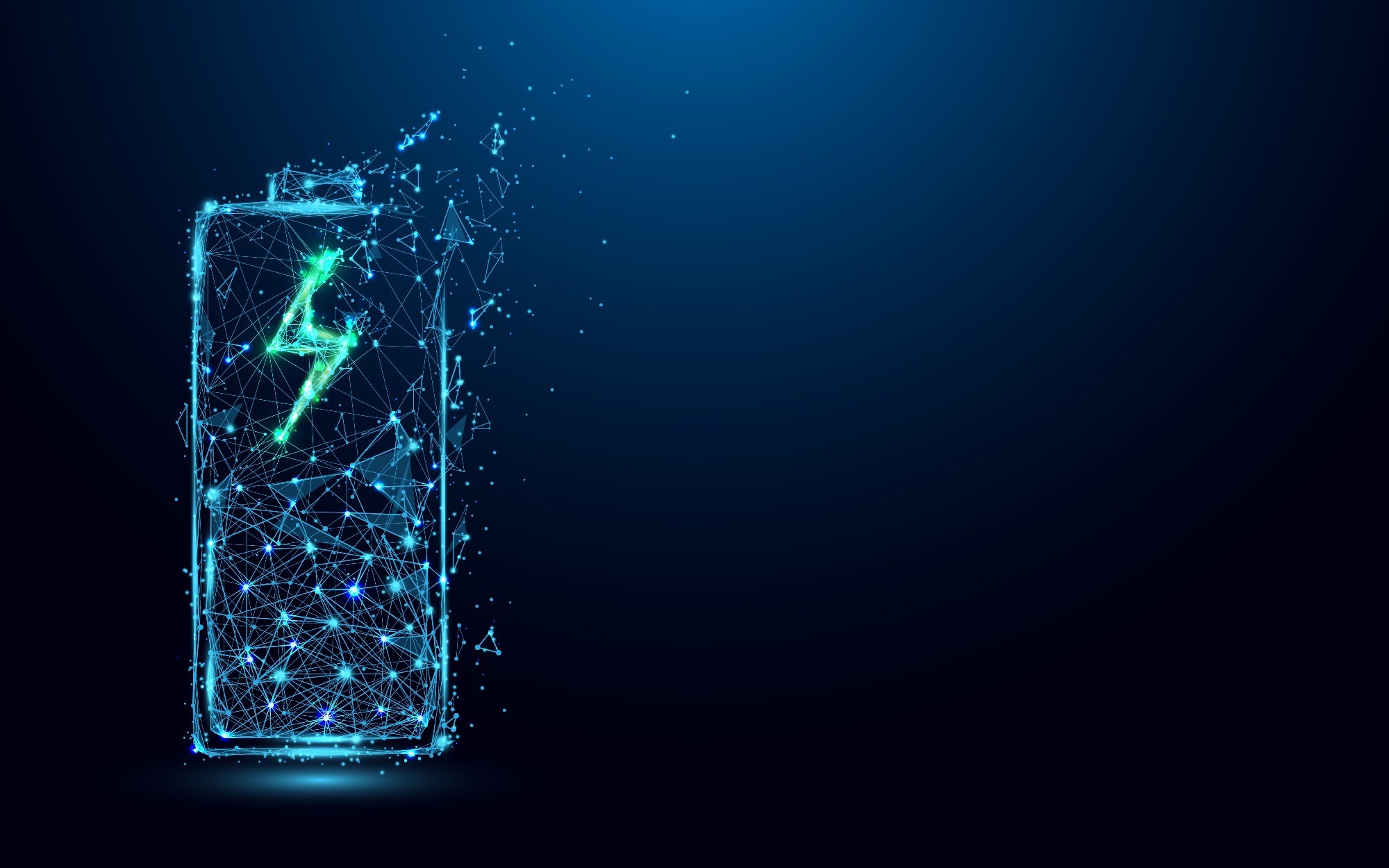
Image credit: Illus_Man/Shutterstock.com
How does vibrational spectroscopy work?
Vibrational spectroscopy probes how light interacts with the vibrational motions within molecules. Fourier transform infrared spectroscopy (FTIR) and Raman spectroscopy are complementary techniques. Both probe different types of molecular vibrations. FTIR probes asymmetric vibrations, while Raman spectroscopy focuses on symmetric vibrations.
Symmetric vibrations (like carbon-carbon) appear more intensely in the Raman spectrum than in the infrared spectrum. Asymmetric vibrations between carbons and oxygens, where there is a dipole moment that is polar, appear stronger in the infrared spectrum than in the Raman spectrum.
How is vibrational spectroscopy useful in battery research?
There are many ways to utilize vibrational spectroscopy, so perhaps an example is the best place to start. If we look at electrode materials, there is a need for advancements in the chemistry and materials used for electrodes.
The battery electrodes are an incredibly important component of the electrochemical cell. In commercially available lithium-ion batteries, the traditional electrode materials have nearly exhausted their capacity to provide improved performance and cost reduction. Researchers are exploring new materials that can allow battery technology to leap into the next generation of capacity and applications.
The Thermo Scientific Raman and FTIR instruments are used to study the composition and electrochemical properties of new candidate materials for battery electrodes using Raman instruments, including the DXR3 Raman Microscope, the DXR3 Raman Flex Spectrometer, or the Nicolet iS50 FTIR Spectrometer can be configured with a wide range of sampling accessories.
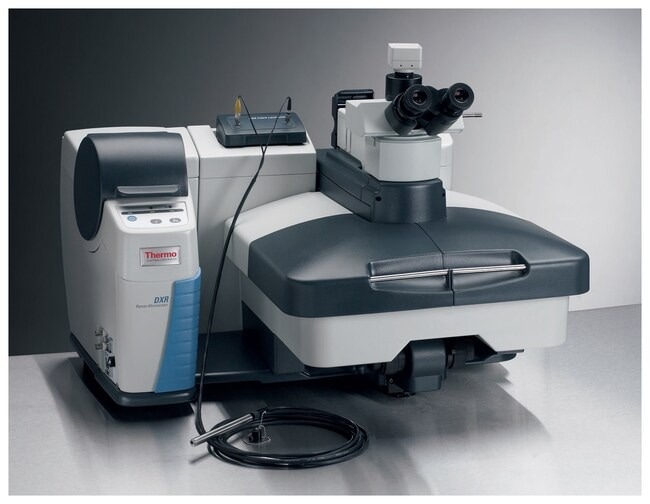
DXR3 Raman Microscope. Image credit: Thermo Fisher Scientific
Why are new materials needed in battery technology?
For cathode materials, the conventional materials used pose challenges, such as high cost, safety and toxicity issues, and issues associated with capacity fade. There is a considerable opportunity for discovery in terms of cathode materials. For example, researchers are looking at nanomaterials to develop better cathode materials, utilizing their increased surface area.
Regarding anodes, graphitic materials are commonly used, but their theoretical capacity is limited for graphite. One very attractive material is silicon, due to its very high theoretical capacity. The challenge of implementing silicon as an anode material lies in its tendency to undergo expansion during battery cycling. These volume changes can result in degradation. This is an important opportunity for research advancements.
Has vibrational spectroscopy proved useful in the research into cathode materials?
Understanding electrode chemistry and its overall impact on the cell is critical for simultaneously measuring the composition, morphology, and chemistry occurring within electrodes. Vibrational spectroscopy is extremely well suited for meeting that need.
Can you share some examples illustrating the capabilities of vibrational spectroscopy?
Vibrational spectroscopy can be used in graphite electrode analysis. Raman spectroscopy is a wonderful carbon analyzer because of those carbon-carbon bonds with symmetric vibrations.
Raman spectroscopy can also be used to probe the broader morphology of a material and can distinguish between the different allotropes of carbon, such as graphite, single-layered graphene, carbon nanotubes, buckyballs, and more.
The spectra of graphite and graphene differ from one another, and the relative intensities of key characteristic peaks change. Defects in the material can also be identified through the emergence of a defect peak in the Raman spectra.
Raman microscopy can produce color-coded chemical maps of electrode materials that distinguish between single-layer graphene, defect regions, and creases resulting from the transfer process. There is a wealth of information that can be acquired quickly and easily, without destroying the sample, by probing these carbon materials with Raman spectroscopy.
Another example is silicon anode studies. Researchers are trying to address the challenges of silicon by looking at nanocrystalline silicon. In a Raman spectrum of silicon, the peak changes very dramatically, indicating changes in crystallinity from highly structured to amorphous. The Raman map image identifies the distribution of those different silicon types on the active area of the sample type.
When aiming to advance current technology, cathode materials also present numerous challenges. With vibrational spectroscopy, nanomaterials and other composite materials can be screened for morphologies, structure, and stoichiometry.
Further work has also been conducted utilizing an accessory called the Jackfish cell by PIKE Technologies, which fits right into the Nicolet iS50 FTIR Spectrometer. This simple technique, named surface-enhanced infrared absorption spectroscopy, provides sub-mono-layer information about the chemistry and chemical structure at the electrode surface.
What are the challenges of current electrolyte materials and what is the potential of these materials in the future?
The electrolyte is a very critical part of the overall battery cell. There is a need to move away from conventional liquid electrolytes as they pose risks of leakage and limit the applications for which batteries can be employed.
There is a growing momentum to transition from liquid electrolytes to solid or semi-solid electrolytes. This creates the opportunity for flexible batteries, adaptable for use in clothing and various other applications.
Solid polymer electrolytes, metal-organic framework materials, covalent organic framework materials, and hydrogels are solid electrolyte materials of interest.
How is vibrational spectroscopy being used in the research advancing electrolyte materials?
Vibrational spectroscopy is a valuable tool for investigating the structure of electrolyte materials and the charge transfer dynamics in a quick screening manner.
In one example, Raman Spectroscopy was used to study a solid polymer electrolyte. One challenge with solid polymer electrolytes is that they can exhibit reduced ionic conductivity, primarily due to hindrances caused by crystalline regions within the electrolyte structure.
To overcome this, additives and dopants have been introduced to the electrolyte to eliminate or inhibit the formation of those crystalline regions. Raman microscopy will reveal how the additives affect the overall composition and structure of the electrolyte.
Are there other applications of this device that have not been discussed?
There are examples of in operando studies that can be done utilizing another accessory. An EL-Cell is a sampling cell that allows the construction of an electrochemical cell within this sampling accessory.
Placing it under the objective in the Raman microscope enables the visualization of both the chemical and morphological changes occurring at the electrode during battery cycling.
Are there analytical techniques that can be combined, and if so, how is that beneficial?
The Nicolet iS50R FTIR Spectrometer, can be coupled with other analytical techniques to extract further information from a single experiment. This includes coupling with gas chromatography, thermogravimetric analysis, or mass spectrometry. Any permutation of these is supported and being conducted currently.
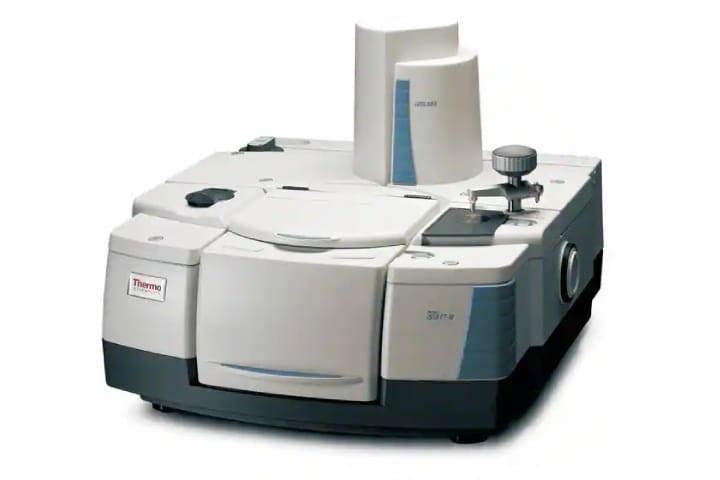
Thermo Scientific Nicolet iS50 FTIR Spectrometer. Image Credit: Thermo Fisher Scientific
Coupling all this information together generates a complete picture of the chemical dynamics of a battery, enabling the required advancement into next-generation technologies.
About Lena Woodis
Dr. Lena Woodis is the Associate Product Manager for research FTIR at Thermo Fisher Scientific, primarily supporting the Nicolet iS50 and Nicolet iS50R high-end research products. Lena has over 15 years of experience in chemical instrumentation and vibrational spectroscopy.
She holds a Ph.D. in physical chemistry from the University of Wisconsin—Madison, for which she developed ultrafast coherent multidimensional spectroscopic techniques. Following graduate study, Lena continued in academia for 10+ years as a chemistry professor prior to transitioning to product management.
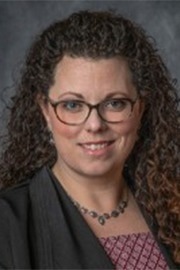
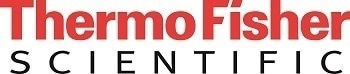
This information has been sourced, reviewed, and adapted from materials provided by Thermo Fisher Scientific – Materials & Structural Analysis.
For more information on this source, please visit Thermo Fisher Scientific – Materials & Structural Analysis.
Disclaimer: The views expressed here are those of the interviewee and do not necessarily represent the views of AZoM.com Limited (T/A) AZoNetwork, the owner and operator of this website. This disclaimer forms part of the Terms and Conditions of use of this website.