Low-temperature plasma polymerization has been proven to be an economic and versatile method to deposit polymer coatings.1
This technique is clean, free of solvents, and can deposit coatings onto a variety of substrate materials. The plasma polymerization process stands out from conventional polymerization through several key features:2
- The polymers formed do not have a recognizable repeat unit
- Polymer properties largely depend on the conditions of polymerization and the initial monomer structure
- A conventional polymerizable functional group, such as a double bond, is not required by potential monomers
The chemistry of plasma polymerization is a non-equilibrium, non-thermal process, and the electron temperature in non-equilibrium plasmas is several orders of magnitude greater than the gas temperature.2 Additionally, chemical reactions can occur at lower temperatures than those possible under thermal conditions.
However, a major limitation of the plasma polymerization technique is that reactive processes, such as cross-linking reactions, UV damage, and ion bombardment2 in the plasma, form polymer networks with complex structures. These polymer networks bear little resemblance to the precursor molecule. As a result, films containing high levels of chemical specificity are very difficult to produce.
Variation of experimental parameters, such as input power,3,4 nature of substrate,3,5 gas composition and pressure,5,6 substrate temperature,6-9 substrate position,10 reactor dimensions11 and so on, provide some level of control over the stoichiometry. For instance, the retention of functional groups in many of the systems studied increases when the power supplied to the plasma is reduced. 3 Yet, the overall selectivity is likely to remain fairly poor.
This study reports on the sputter depth profiling of acrylic acid plasma polymer, which was deposited with different degrees of cross-linking.
As shown in Figure 1, the acrylic acid monomer molecule contains a double bond and an acid group. The degree of cross-linking can be understood by the amount of acid group retained in the polymer film, as measured by XPS.
It must be noted that no attempt has been made in this study to differentiate between the ester group (COOC) and the carboxylic acid group (COOH). It was found that both chemical groups possess identical chemical shifts in the XPS spectrum.
.jpg)
Figure 1. Acrylic acid monomer molecule
Experimental and Results
Kratos’ Minibeam 6 multi-mode Ar gas cluster ion source (GCIS) was used for sputter depth profiling of thin plasma polymer films (Figure 2).
This ion source can produce massive Ar clusters of up to 3000 atoms per cluster ion, which are accelerated by potentials up to 20 keV for sputter depth profiling of organic materials. Large cluster ions, unlike monatomic ions, do not penetrate deeply into the subsurface region.
The impact produces energy which is dissipated within the first few nanometres of the surface of the sample.12 Since all the atoms in the cluster share the total ion energy, the energy per projectile atom (called the partition energy) can be as low as a few electron volts which results in gentle removal of the top few nanometres of the surface without causing any major chemical damage to the underlying material.
.jpg)
Figure 2. Kratos’ Minibeam 6 multi-mode gas cluster ion source
Figure 3 illustrates a schematic of the GCIS. The ion source can be used in several modes such as — monatomic Ar+ mode for metals and other inorganic samples; massive Arn+ cluster mode for depth profiling of organic samples; low energy He+ mode for ion scattering spectroscopy; and medium cluster, high energy mode for sputtering inorganic oxides.
In cluster mode, the isentropic adiabatic cooling of Ar gas results in the formation of large Ar clusters as the Ar gas expands from high pressure into the vacuum of the source region via a de Laval nozzle. The Arn clusters, thus formed, are subsequently ionized by electron impact and then accelerated along the ion column.
The sampled range of cluster size is narrowed by using a Wien velocity filter. The ions are accelerated up to a maximum of 20 kV, focused, and rastered across the sample surface.
.jpg)
Figure 3. Schematic of the Minibeam 6 multi-mode gas cluster ion source.
A standard multilayer polymer sample was profiled before the plasma polymers to characterize the performance of the ion source. The sample, which was supplied by the National Physics Laboratory (UK) as part of the EURAMET project, contained alternating layers of NPB/ Alq3. Figure 4 shows the schematic of the layer structure.
NPB is a polymer that contains H and C. Alq3 [Al(C9H6NO) 3] delta layers have 2.9% Al which can be used as a marker to describe the sample’s multilayer structure.
.jpg)
Figure 4. EMRP NPB/ Alq3 multilayer sample
5 keV Ar1000+ ions were used to etch the multilayer. Figure 5 illustrates the measured elemental composition of the sample. The etch rate, under these conditions, was 28 nm/minute and the ion dose to the interface with the Si substrate was 8 x 1014 ions/cm2. The Alq3 layers were suitably resolved and showed good interfacial resolution.
.jpg)
Figure 5. Alq3 / NPB Multilayer depth profile. O, C and Si concentration are plotted against the left hand Y axis while the Al concentration is plotted on the right hand Y axis.
The GCIS was used to profile three acrylic acid plasma polymers (ppAA), which were deposited under the following conditions:
- Sample 1: 10 W plasma discharge power, sample positioned 10 cm from the electrode
- Sample 2: 10 W plasma discharge power, sample positioned 5 cm from the electrode
- Sample 3: 50 W plasma discharge power, sample positioned 5 cm from the electrode
Before profiling, spectroscopic ellipsometry was used to measure the sample thicknesses of 46, 76 and 83 nm for samples 1, 2, and 3, respectively. C 1s XPS spectra of the as-received surface show the various levels of acid group retention (Figure 6).
.jpg)
Figure 6. C 1s spectra of the ppAA as introduced surface.
As predicted, the largest concentration of surface acid groups was retained by sample 1 because this polymer was deposited under the most benign plasma conditions: (low power, remote from the electrode). However, sample 3 retained the least amount of acid groups; (high power, close to the electrode).
The ppAAs were sputter profiled using Ar1000 clusters at acceleration energies of 5, 10 and 20 keV. This rendered partition energies of 5, 10 and 20 eV for each atom. In Figure 7, profile results are plotted as yield volume (amount of material removed per cluster ion) against the partition energy (average energy per atom).
.jpg)
Figure 7. Depth profile of ppAA at different beam conditions.
When the partition energy increases, the yield volume per ion also increases in a pseudo linear fashion over the limited energy range studied. In all the cases, it was observed that the more cross-linked ppAA (sample 3) possessed a lower yield volume for a given ion energy.
The chemical concentration of the ppAA, for all samples and at all beam energies, did not differ through the film. This is illustrated for sample 1 by a plot of the O concentration versus depth in Figure 8.
.jpg)
Figure 8. Sample 1. O concentration as a function of depth at 3 different beam acceleration voltages.
Similarly, the films’ C chemistry remained constant across the profiles at all beam energies. This is again shown for sample 1 by a plot of the C chemistry versus depth at 5 eV partition energy in Figure 9.
.jpg)
Figure 9. C chemistry as a function of depth for sample 1 at a partition energy of 5 eV.
Figure 10 shows an overlay of the C 1s spectra of sample 1 from the surface and after an ion dose of 8 x 1013 ions/cm2 (nearing the interface with the Si substrate), demonstrating that during the profile, the ppAA is not chemically affected.
.jpg)
Figure 10. Overlay of C 1s spectra of sample 1 from the surface (red) and after an ion dose of 8 x 1013 ions/cm2 (nearing the interface with the Si substrate).
Conclusion
This article shows that the multi-mode Ar gas cluster ion source can be effectively used for sputter depth profiling of a range of organic samples with different chemical structures.
In this analysis, it has been demonstrated that the ion yield depends on the degree of cross-linking, particularly for acrylic acid plasma polymers. Moreover, the removal of surface layers by massive Ar gas clusters did not change the chemical nature of the sample. The ion yield was also dependent on the beam’s acceleration voltage.
Download the Application Note for More Information
Acknowledgments
Kratos would like to thank Prof. Sally McArthur of the Faculty of Science, Engineering and Technology, Swinburne University of Technology for providing the acrylic acid plasma polymer samples.
Kratos would also like to thank Alex Shard and Steve Spenser, NPL, for providing the Alq3 calibration standard.
References and Further Reading
- Yasuda, H. Plasma Polymerization; Academic Press, Inc.: London, 1985.
- Grill, A. Cold Plasmas in Materials Technology; IEEE Press: New Jersey, 1994.
- d’Agostino, R.; Favia, P.; Fracassi, F. J. Polym. Sci.: Part A: Polymer Chemistry 1990, 28, 3387.
- Alexander, M. R.; Duc, T. M. J. Mater. Chem. 1998, 8(4), 937.
- Clark, D. T.; Shuttleworth, D. J. Polym. Sci.: Polym. Chem. Ed.1980, 18, 27.
- d’Agostino, R.; Cramarossa, F.; Illuzzi, F. J. Appl. Phys. 1987, 61(8, 15 April), 2754.
- López, G. P.; Ratner, B. D. J. Polym. Sci.: Part A: Polymer Chemistry 1992, 30, 2415.
- López, G. P.; Ratner, B. D. J. Appl. Polym. Sci.: Appl. Polym. Sym 1990, 46, 493.
- Yasuda, H.; Wang, C. R. J. Polym. Sci.: Polym. Chem. Ed. 1985, 23, 87.
- Shard, A. G.; Munro, H. S.; Badyal, J. P. S. Polym. Chem. 1991, 31, 152.
- Kim, H. Y.; Yasuda, H. K. J. Vac. Sci. Technol. A 1997, A15(4), 1837.
- Gillen, G.; Roberson, S.; Rapid Comm. Mass Spectrom. 1998, 12, 1303-1312.
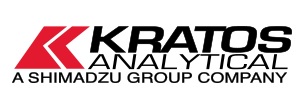
This information has been sourced, reviewed and adapted from materials provided by Kratos Analytical Ltd.
For more information on this source, please visit Kratos Analytical Ltd.