Efficient 1.5 W CW and 9 mJ quasi-CW TEM00 mode operation of a compact diode-laser-pumped 2.94 µm Er:YAG laser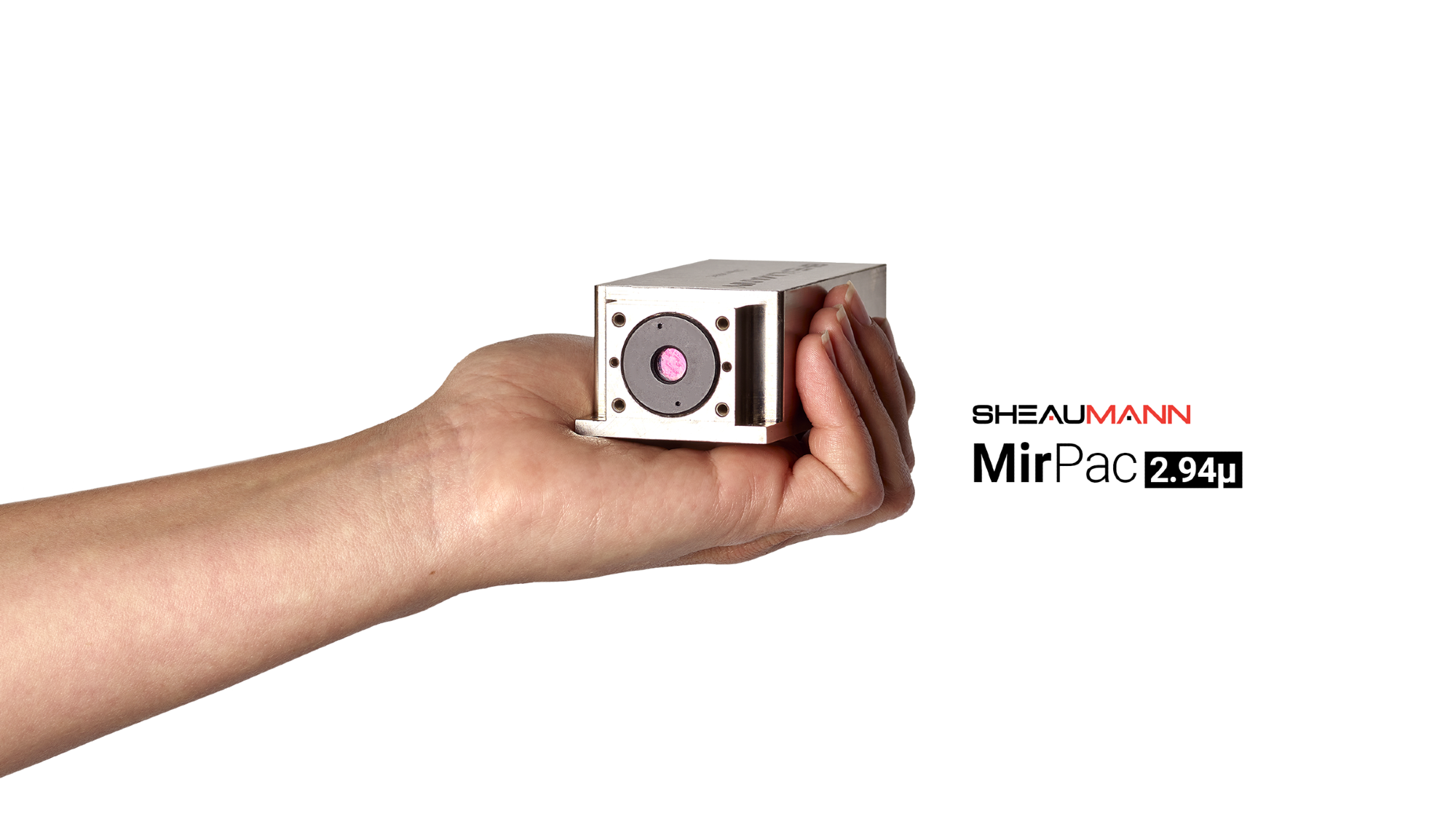
Sheaumann has developed the first commercially available diode-laser-pumped continuously operating (CW) 1.5 W, 2.94 µm Er:YAG laser. This laser operates in the end-pumped microlaser chip configuration, which was first demonstrated by Chen et al.1 and was later extended to other Er3+-doped garnets by Dinerman and Moulton.2
The development of long-lasting, higher brightness and power diode pump laser sources has led to this technology becoming commercially viable.
A high brightness pumping source is essential, due to the energy level dynamics of a laser. Figure 1 is an energy level diagram, showing that the 6.4 ms lifetime of the lower laser level (4I13/2) is significantly longer than the 0.1 ms lifetime of the upper laser level (4I11/2). This difference would result in self-saturation under ordinary circumstances.
![A simplified energy level diagram for Er:YAG showing the pump absorption and laser transitions. Cooperative upconversion between pairs of Er3+ ions provides greater than unity quantum efficiency and overcomesbottlenecking in the 4I13/2 lower laser level. This diagram was reproduced from Georgescu and Toma [4].](https://d12oja0ew7x0i8.cloudfront.net/images/Article_Images/ImageForArticle_20570_4439227517524317248.png)
Figure 1. A simplified energy level diagram for Er:YAG showing the pump absorption and laser transitions. Cooperative upconversion between pairs of Er3+ ions provides greater than unity quantum efficiency and overcomes bottlenecking in the 4I13/2 lower laser level. This diagram was reproduced from Georgescu and Toma [4]. Image Credit: Sheaumann Laser, Inc.
At high Er3+ concentrations under intense pumping, however, cooperative upconversion between neighboring Er3+ ions is seen to deplete the 4I13/2 level of one ion while at the same time providing a pumping path to the upper laser level via the 4I9/2 energy level (column (a), Figure 1).
This effect stops the laser from self-terminating, while simultaneously providing almost two photons at the laser wavelength for each absorbed pump photon population. This results in greater than unity quantum efficiency.1,3,4 Upconversion from the 4I11/2 energy level (column (b), Figure 1) along with cross-relaxation from the 4S3/2 energy level (column (c), Figure 1) have also been found to have a significant impact on 2.94 µm Er:YAG laser dynamics.4
Flashlamp pumped 2.94 µm Er:YAG and Er:YSGG lasers are primarily employed in hard and soft tissue dental procedures. There has also been recent interest in the application of lower energy quasi-cw diode-laser-pumped devices in micro-surgical applications.5
This article explores the performance of a 1.0 W cw 2.94 µm Er:YAG laser (specifically Sheaumann’s MIR-Pac laser), as well as its quasi-cw characteristics. When utilized as an OPO pumping source, this device’s current incarnation has great potential for use in soft tissue micro-surgical applications as well as other application areas like spectroscopy.
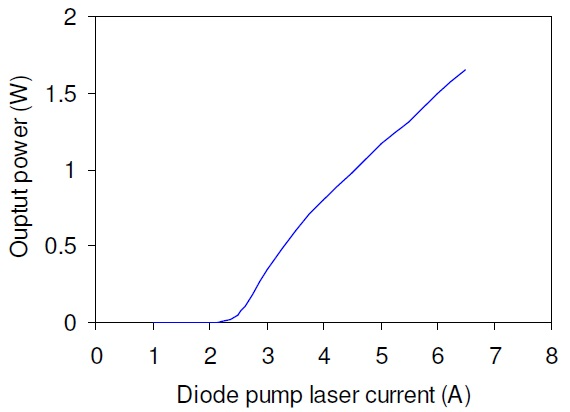
Figure 2. CW output power of a 2.94-μm MIR-Pac laser versus the diode pump laser drive current. Image Credit: Sheaumann Laser, Inc.
CW Laser Performance
When working in cw operating mode, the MIR-Pac laser has been specified for operation at a maximum of 1.0 W of output power (Figure 2) in an M2<1.2, TEM00 mode beam (Figure 3) with a standard beam divergence of 17 mrad.
Recent advances have resulted in the generation of up 1.5 W output power. This is limited by the onset of thermal rollover, which is believed to be related to thermal lensing in the microlaser chip.
Work is currently in progress, exploring the use of a short laser resonator to independently control mode size and resonator stability. This would, ideally, facilitate efficient operation at higher output powers.
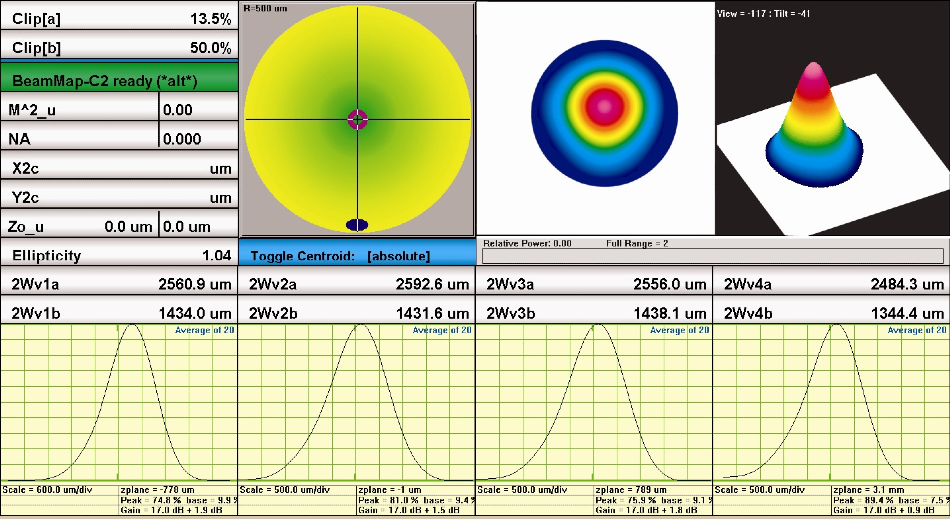
Figure 3. Typical TEM00 output beam data for the 2.94-μm MIR-Pac laser. Image Credit: Sheaumann Laser, Inc.
Quasi-CW Laser Performance
The 2.94 µm Er:YAG laser is optically pumped using a diode laser. This diode laser may be pulsed or operated continuously using different drive current waveforms.
Driving the diode laser via a rectangular current pulse with rise times <10 µs will lead to the corresponding optical pump pulse efficiently creating a population inversion exceeding the lasing threshold. Nominally rectangular laser pulses with durations from <0.1 ms to a large fraction of a second may be generated. This is known as quasi-cw operation, due to the pulse durations being long enough for the laser output to attain a steady-state.
In this particular mode of operation, the laser can be operated at peak powers, proportionally higher than the average laser power operating limit by a factor that is the inverse of the duty cycle of the quasi-cw laser pulse train. Current implementation of this technique is limited by the diode pump laser’s maximum peak power of approximately 10 W. Introduction of the next generation device will see this increase, however.
Meister et al.5 successfully demonstrated the generation of 15.7 mJ in 2.5 ms pulses from a quasi-cw diode-laser-pumped Er:YSGG laser that had been designed for use in soft tissue microsurgical applications. This compares favorably to Sheaumann’s 6 mJ in 2.5 ms data, which is presented below.
Meister et al. also provide a thorough review of other quasi-cw diode-laser-pumped 3-micron Er-lasers capable of generating 30 mJ energies, but these are not able to be optimized for efficient operation at lower pulse energies. Furthermore, these lasers are not cost-effective due to their utilization of stacked arrays of diode laser bars.
Figure 4 displays a standard quasi-cw Er:YAG laser pulse that has been generated via pumping with a 0.5 ms diode laser pulse at a 1000 Hz repetition rate.
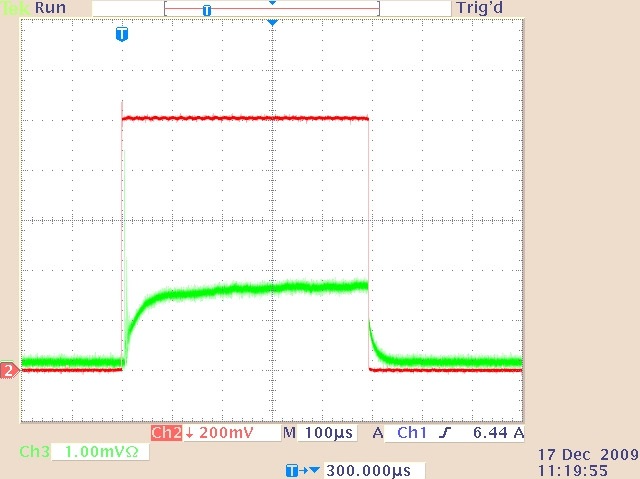
Figure 4. The quasi-cw laser output (lower trace) and drive current waveform (upper trace) for a 0.5-ms duration, 10 A current pulse at a 1000 Hz repetition rate. Image Credit: Sheaumann Laser, Inc.
A series of gain-switched pulses are emitted at the onset of lasing. These then transition into the established damped relaxation oscillation (Figure 5) condition followed by oscillation free quasi-cw laser operation that is immediately terminated after the pump pulse has been extinguished.
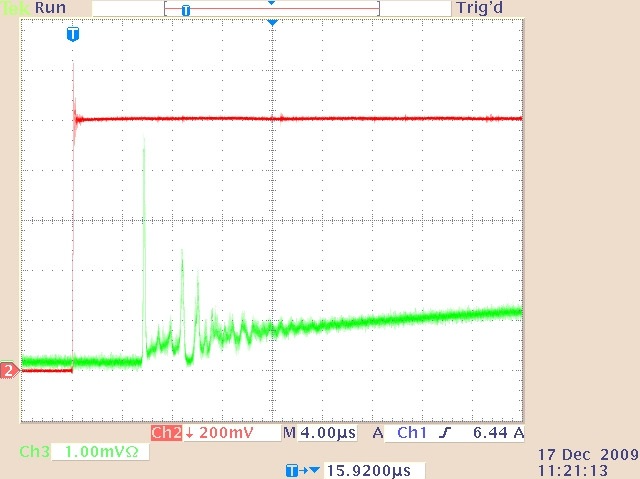
Figure 5. The quasi-cw laser output (lower trace) and drive current waveform (upper trace) showing the relaxation oscillations at the beginning of the pulse shown in Figure 4. Image Credit: Sheaumann Laser, Inc.
A 6 µs, build up time delay can be measured from the beginning of the pump pulse to the lasing threshold. This build up time delay will become shorter with higher peak pump powers, while for the generation of millisecond duration pulses it is almost insignificant. The 100 µs transition to the stable quasi-cw is more significant, however, when generating sub-millisecond pulses.
Relaxation oscillation damping time (τ0) of an ideal four level laser6 is given by the following formula:
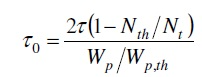
Here, the characteristic lifetime of the upper laser level (τ) is modified by the ratio of the population inversion density at threshold to the total number of active ion sites in the pumped region of the gain medium, Nth Nt, and the number of times the optical pumping rate is seen to exceed the lasing threshold, Wp Wp,th.
Pumping harder clearly shortens the relaxation oscillation damping time. This fundamental principle still applies to the 2.94-µm Er:YAG laser, despite its energy level dynamics being far more complex than those of the ideal four level laser.
Pumping the Er:YAG laser just below its lasing threshold before the main pumping pulse can shorten the relaxation damping time, almost fully eliminating the build up time delay (Figure 6).
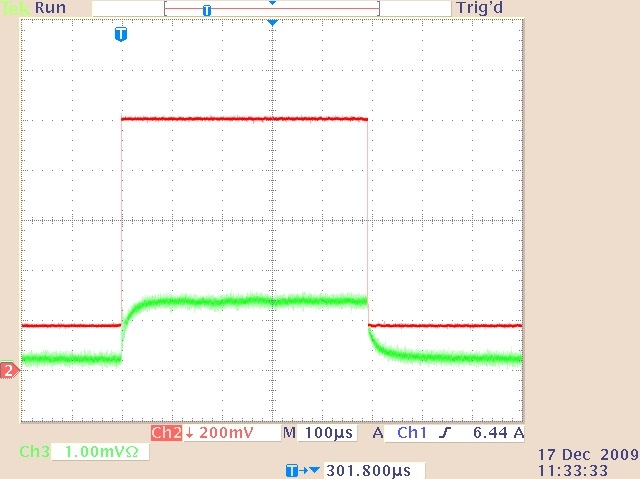
Figure 6. The quasi-cw laser output (lower trace) and drive current waveform (upper trace) for a 1.0-ms duration, 8.2 A current pulse superimposed on a 1.8 A continuous bias current at a 1000 Hz repetition rate. Image Credit: Sheaumann Laser, Inc.
The most ideal pulsed drive current for the laser’s quasi-cw operation is comprised of a continuous bias current set to 80% to 90% of the continuous lasing threshold value (typically 2 A), along with a larger pulsed drive current.
The peak drive current may even be as high as the 10 A maximum permitted drive current of the diode pump laser. However, this has an additional constraint in that the duty cycle corrected average 2.94-µm laser output power must not exceed the device’s specified maximum operating power.
Following an optical pump pulse, most quasi-cw lasers will turn off with a monotonic decay in intensity. Sheaumann’s researchers observed this behavior when they completely switched off diode pump laser at the end of the pumping pulse. However, when the diode pump laser was operated continuously between the pumping pulses (operating at just under the Er:YAG laser threshold) a series of decaying relaxation oscillations were observed (Figure 7) at the end of the Er:YAG laser pulse.
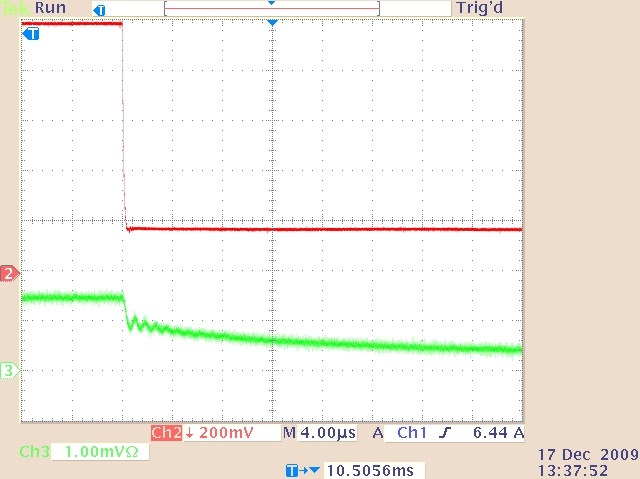
Figure 7. The quasi-cw laser output (lower trace) and drive current waveform (upper trace) showing the relaxation oscillation decay at the end of the laser pulse shown in Figure 6. Image Credit: Sheaumann Laser, Inc.
These oscillations are believed to be a unique feature of the laser dynamic response, occurring as a result of the continued and slowly decaying optical pumping of the upper laser level via the upconversion pathway providing phase delayed feedback.
Ultimately, the Er:YAG laser’s pulsed peak power capability is limited by the same thermal fracture mechanism as it is in continuous operation. For the current product this is specified at 1 W, but recent device improvements now allow operation up to 1.5 W (Figure 1).
Therefore, the corresponding recommended peak power limit for quasi-continuous operation at a duty cycle of 10% - 1 ms pulses at a repetition rate of 100 Hz - would be >10 W. In practice however, the pump diode laser included in the standard 2.94 µm MIR-Pac Er:YAG laser product is limited to a maximum drive current of 10 A, which generally corresponds to peak powers up to 2.5 W.
Data shown in Figures 8 and 9 shows the generation of up to 2.5 W peak powers in quasi-cw operation, operating at the maximum drive current limit to generate millisecond regime pulses at pulse repetition rates. Here, maximum pulse energies of 9 mJ were generated for 5 ms pulses at 1.8 W of peak power. The average power limit was only attained at duty cycles of around 50% and pulse peak powers as high as 2 W. Corresponding pulse energies were 1 mJ at 1 kHz.
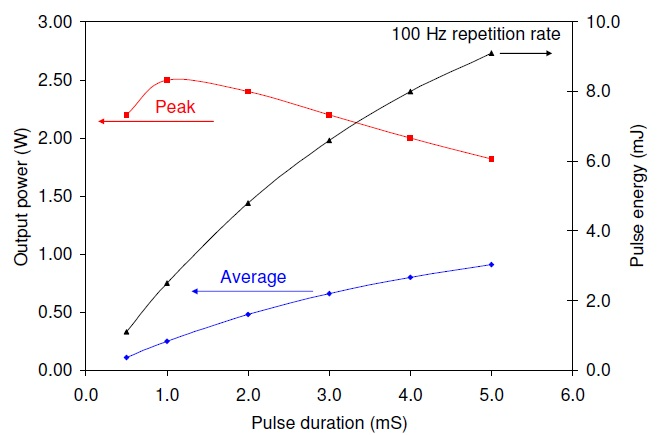
Figure 8. The quasi-cw laser performance at a fixed pulse repetition rate of 100 Hz for 8.2 A drive current pulses superimposed on a 1.8 A continuous bias current. Image Credit: Sheaumann Laser, Inc.
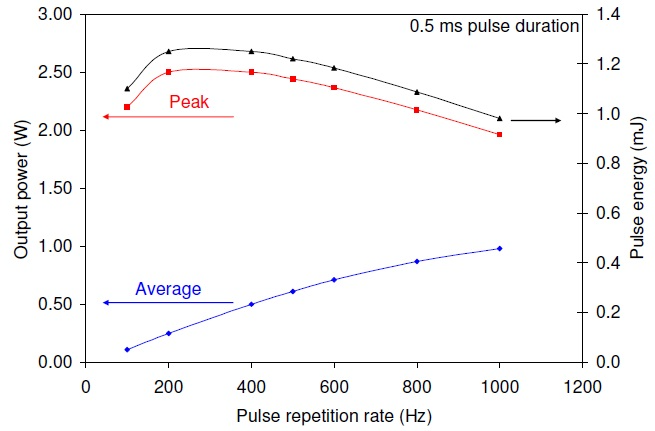
Figure 9. The quasi-cw laser performance at a pulse duration of 0.5 ms for 8.2 A drive current pulses superimposed on a 1.8 A continuous bias current. Image Credit: Sheaumann Laser, Inc.
When working at low duty cycles and with extended intervals between laser pulses, transient thermal lensing leads to unwanted spatial mode modulation. Operating the Er:YAG laser near to its threshold, but not lasing, between laser pulses can help to avoid this problem by introducing a substantial fraction of the thermal lens experienced under lasing conditions.
This issue is illustrated in Figure 10, where the pulse’s rising edge walks through a series of relaxation oscillation events that indicate multiple spatial mode evolution. Figure 11 displays the elimination of this problem.
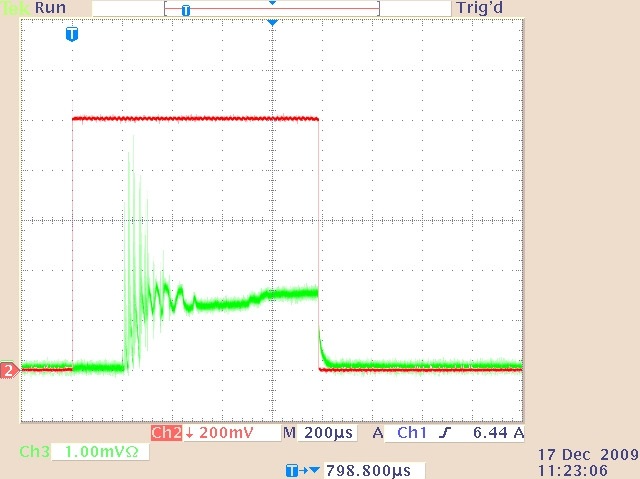
Figure 10. The quasi-cw laser output (lower trace) and drive current waveform (upper trace) for 0.5-ms duration. 10 A drive current pulse. Image Credit: Sheaumann Laser, Inc.
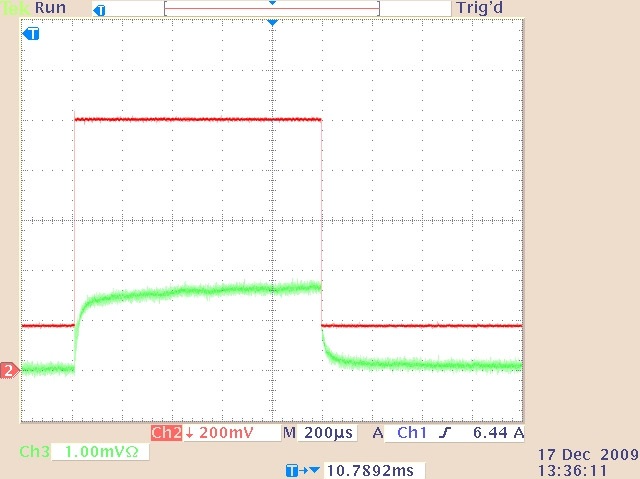
Figure 11. The quasi-cw laser output (lower trace) and drive current waveform (upper trace) for 0.5-ms duration. 8.2 A drive current pulse superimposed on a 1.8 A continuous bias current. Image Credit: Sheaumann Laser, Inc.
This data was taken from an Er:YAG laser in a hermetically sealed windowed package (Figure 12). Sheaumann has also recently tested fiber-coupled versions of this laser package with 0.22 NA ZBLAN (IRphotonics) fibers. These fibers ranged in core diameter from 150 µm to 450 µm.
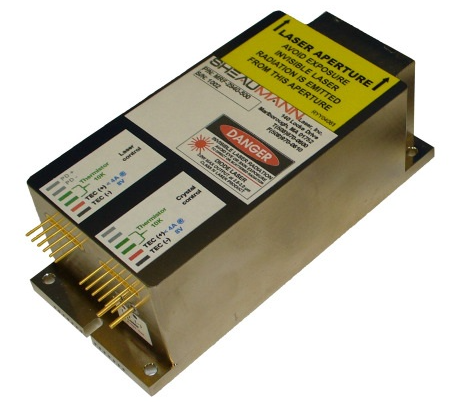
Figure 12. Direct Beam Output. Image Credit: Sheaumann Laser, Inc.
Fiber-coupled output powers of 1.1 W were acquired for 1.3 W of power incident on the input face of 1.5 m long fibers of every core size. After the output fiber face Fresnel loss had been accounted for, power coupling efficiency was estimated to be >90% (Figure 13).
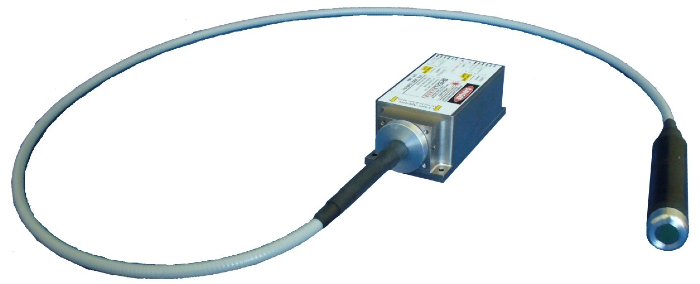
Figure 13. Fiber Coupled Version. Image Credit: Sheaumann Laser, Inc.
Sheaumann has also developed an effective diode-laser-pumped 1.5 W, cw 2.94 µm Er:YAG laser possessing a TEM00 output beam in a hermetically sealed windowed package. This features a fiber-coupled output beam delivery option providing 1.1 W from 150 µm to 450 µm core diameters along with 0.22 NA ZBLAN (IRphotonics) optical fibers. This new laser has been successfully operated in the quasi-cw mode to generate pulse energies as high as 10 mJ in a 5 ms pulse at a 100 Hz repetition rate.
1 mJ pulses can also be generated at repetition rates from a single-shot to 1 kHz. These millisecond duration laser pulses have high beam quality and are ideal for applications requiring controlled heat deposition within small volumes of material. Because of the operating wavelength, this generally involves materials with some water content.
Sheaumann is currently working to increase the cw laser output power to several Watts by employing a longer traditional laser resonator in place of the microlaser chip. Work is also being undertaken to scale the pulse energy and peak power in the quasi-cw regime of operation through the use of higher peak power pump laser diodes and the current Er:YAG microlaser chip. The goal of this work is to be able to generate >5 mJ in 1 ms.
References
- Chen, D., Fincher, C.L., Todd, S.R., Vernon, F.L. and Fields, R.A., “Diode-pumped 1-W continuous-wave Er:YAG 3-µm laser,” Opt. Lett., 24, 385 (1999).
- Dinerman, B.J. and Moulton, P.F., “3-µm cw laser operation in erbium-doped YSGG, GGG and YAG,” Opt. Lett., 19, 1143 (1994).
- Stoneman, R.C. and Estrowitz, L., “Efficient resonantly pumped 2.8-µm Er3+:GSGG laser,” Opt. Lett., 17, 816 (1992).
- Georgescu, S. and Toma, O., “Er:YAG three-micron laser: performances and limits,” IEEE. Sel. Top. Quant. Electron., 11, 682 (2005).
- Meister, J., Franzen, R., Apel, C. and Gutknecht, N., “Multi-reflection pumping concept for miniaturized diode- pumped solid-state lasers,” Appl. Opt., 43, 5864 (2004).
- Zayhowski, J.J., Welford, D. and Harrison, J., [The Handbook of Photonics; Miniature Solid-State Lasers], CRC Press, (2007).
Acknowledgments
Produced from materials originally authored by John Gary Sousa and Josh Foster from Sheaumann Laser, Inc., and David Welford from Endeavour Laser Technologies.

This information has been sourced, reviewed and adapted from materials provided by Sheaumann Laser, Inc.
For more information on this source, please visit Sheaumann Laser, Inc.