Semiconductor integration with microelectromechanical systems (MEMS) refers to the integration of MEMS devices with integrated circuits (ICs) on a single chip, which results in smaller packages/reduced weight and size, improved performance, and lower instrumentation and packaging costs. This article discusses different semiconductor MEMS fabrication methods and their advancements.
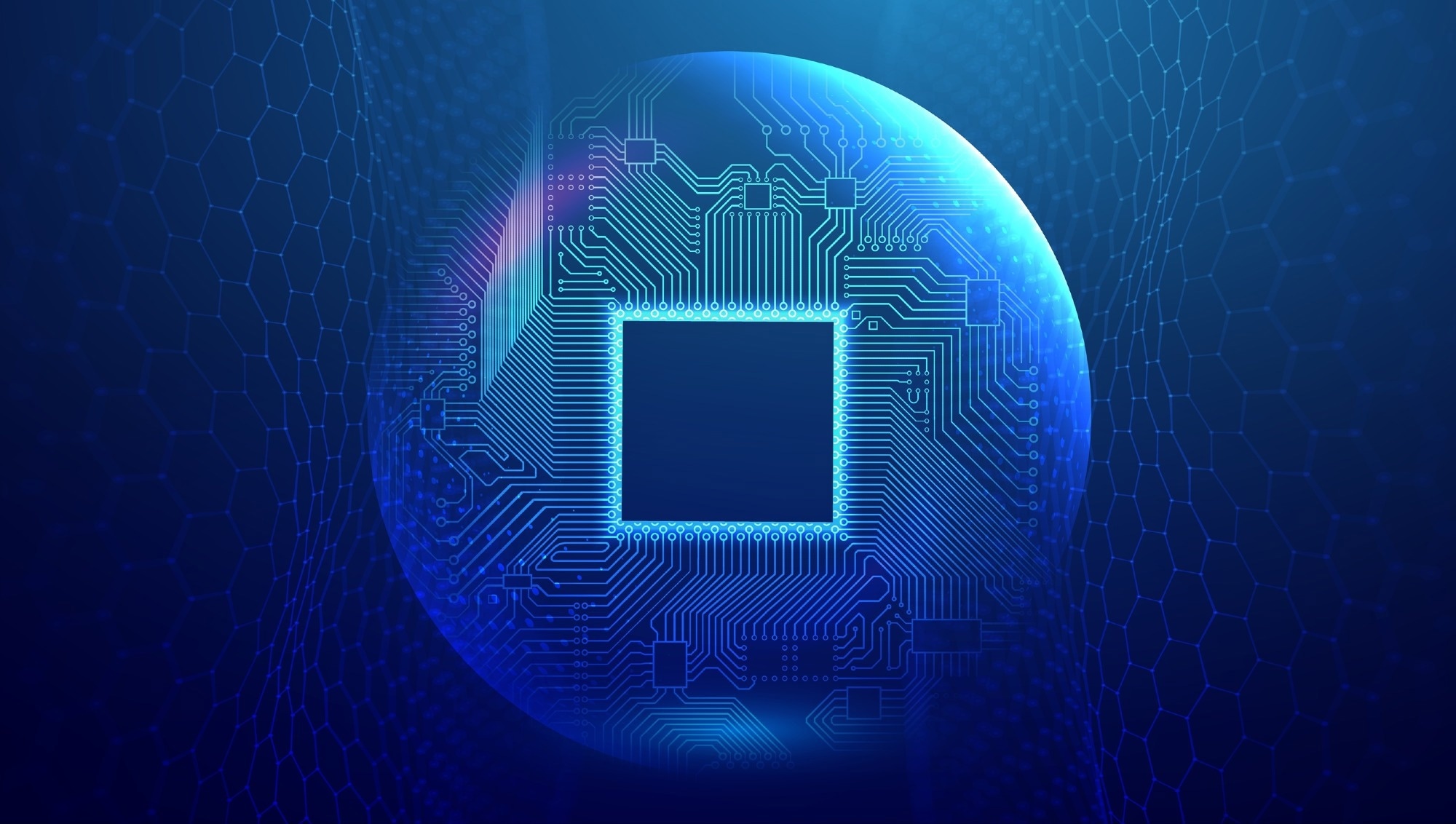
Image Credit: RDVector/Shutterstock.com
Importance of Semiconductor MEMS
MEMS are primarily transducer systems that can control or sense chemical, optical, or physical quantities, such as fluids, acceleration, or radiation. A MEMS device/transducer possesses an electrical interface with the outside world, which is typically realized through ICs that provide the necessary intelligence to enable the device to perform useful functions.
For instance, ICs can provide system testing functions, logic and communication functions, and signal conditioning functions such as analog-to-digital conversion. ICs and MEMS can be integrated using two methods, including hybrid multi-chip integration/ multi-chip solution and system-on-chip (SoC) integration/SoC solution.
In the conventional multi-chip solution approach, both IC and MEMS components are initially synthesized on separate substrates using dedicated IC and MEMS fabrication processes and then hybridized in the final system, while in the more recent SoC solution approach, IC and MEMS components are manufactured on the same substrate using interlaced or consecutive processing schemes.
In recent decades, two-dimensional (2D) integration approaches have been used extensively for the hybrid integration of MEMS and IC technology. In these approaches, the MEMS and IC wafers are designed, fabricated, and tested independently and then separated into discrete chips. The discrete chips are subsequently integrated into multi-chip systems at the package or board level.
Approximately half of all MEMS products, including several microfluidic devices, radio frequency (RF) MEMS, pressure sensors, microphones, gyroscopes, and accelerometers, are currently implemented as multi-chip solutions, while the rest of the MEMS products, including inkjet printheads, infrared bolometer arrays, digital mirror devices, and many pressure sensors, accelerometers, and gyroscopes, are implemented as SoC solutions.
Several semiconductor MEMS products that consist of large transducer arrays in which every transducer is individually operated are primarily implemented as SoC solutions to integrate each MEMS transducer and its associated IC on a single chip.
Semiconductor MEMS through Hybrid Integration
Conventionally, IC and MEMS chips have been packaged separately and then integrated as a system on a printed circuit board (PCB), which led to the development of multi-chip modules. In semiconductor MEMS multi-chip modules, IC and MEMS chips are positioned side-by-side in a common package and interconnected using wire and/or flip-chip bonding at the package level.
Several MEMS and IC integration concepts through flip-chip bonding are utilized in multiple applications, such as micro-opto-electro-mechanical systems, MEMS sensors, and RF-MEMS. Recent concepts for chip-to-package and chip-to-chip interconnections, such as the fan-out wafer-level packaging concept, have been developed based on using thin-film interconnects between embedded chips.
Other chip-to-chip interconnection methods include quilt packaging, in which chips with various functionalities are tiled on the package substrate in close proximity and interconnected using vertical facets protruding from every chip and mechanically flexible interconnects.
Multi-chip modules occupy a lesser area of PCB and possess a significantly reduced signal path length between chips compared to the system-on-board approaches. Thus, this concept is used extensively in research and commercial products.
Specifically, the integration of MEMS chips with application-specific integrated circuits (ASICs) available commercially enables a rapid, simple, and cost-efficient implementation of hybrid semiconductor MEMS systems.
System-in-packages/vertical or stacked multi-chip modules consist of chips attached vertically and interconnected using wire and/or flip-chip bonding either directly or through additional re-distribution layers.
Smaller package footprints/volumes, shorter signal path lengths, and higher integration densities compared to multi-chip modules are the major benefits of these three-dimensional (3D)-stacked approaches, which are employed in commercial products such as pressure sensors.
The wafer-scale package and chip-scale package concepts can yield highly compact packages with footprints similar to the largest chip size involved in the package. Chip-on-MEMS technology is one of the prominent examples of this approach.
System-on-package approaches enable miniaturized and highly integrated system technology at the package level. In these approaches, MEMS and IC devices are integrated with several other basic technologies, ranging from power electronics and optics to wireless components, in a common package.
Thus, low fabrication complexity, modularity, and high flexibility are the key advantages of multi-chip solutions, while thicknesses, large system footprints, and limited integration densities are the major disadvantages.
Semiconductor MEMS through Wafer-level Integration
SoC solutions can be classified as monolithic MEMS and IC integration techniques, in which both the IC and MEMS structures are entirely fabricated on the same substrate, and heterogeneous MEMS and IC integration techniques, in which the IC and MEMS structures are partially or fully prefabricated on separate substrates and then merged on a single substrate.
SoC solutions using monolithic MEMS and IC integration
In monolithic MEMS and IC integration using MEMS-first processing, all processing steps required for a complete MEMS device are performed before the complementary metal-oxide-semiconductor (CMOS) processing to enable the subsequent CMOS integration and fabricate semiconductor MEMS.
These approaches provide favorable MEMS fabrication conditions, such as an extremely high thermal budget, which allows the fabrication of high-performance MEMS structures, such as high-performance MEMS resonators. However, material exposure of the pre-processed MEMS wafers and strict surface planarity requirements are the major disadvantages.
Semiconductor MEMS through monolithic MEMS and IC integration using interleaved MEMS and IC processing is realized using a combination of MEMS processing steps performed after, during, or before CMOS fabrication.
Although these approaches allow the integration of high-performance MEMS devices and materials with CMOS circuits on the same substrate, they require full access to a dedicated custom CMOS production line, which significantly limits the general applicability of this technology.
In monolithic MEMS and IC integration using MEMS-last processing through bulk micromachining, the MEMS structures are fabricated after the completion of the entire CMOS fabrication process.
This approach can be implemented using the existing IC infrastructure, which is a major advantage. However, the limited selection of materials and limited design freedom for the MEMS devices allowed by the CMOS process are the key drawbacks.
Semiconductor MEMS can be realized through monolithic MEMS and IC integration using MEMS-last processing via surface micromachining and layer deposition. In this approach, the MEMS structures are manufactured by micromachining and depositing materials over the completed CMOS wafers.
Although standard CMOS foundries can be used for CMOS wafer fabrication in this approach, the deposition temperature for the MEMS materials has to remain within the allowed CMOS wafer temperature budget/400-450 °C, which makes the technology unsuitable for MEMS devices implemented using high-performance MEMS materials.
SoC Solutions Using Heterogeneous MEMS and IC Integration
Heterogeneous MEMS and IC integration refers to the joining of two or multiple substrates containing partially or fully fabricated MEMS and IC structures to fabricate a heterogeneous SoC solution.
Heterogeneous MEMS and IC integration via formation during layer transfer/via-first processes allows the integration of high-performance MEMS materials with standard CMOS-based IC wafers. However, they often require aligned substrate-to-substrate bonding, which increases process complexity and leads to limitations on the achievable post-bonding alignment accuracies.
Recent Studies
In a study recently published in the 2020 IEEE 33rd International Conference on Micro Electro Mechanical Systems (MEMS), researchers developed an integrated thermoresistive micro calorimetric Flow (TMCF) sensor with µm/s detection limit using the proprietary InvenSense CMOS MEMS technology.
The TMCF sensor demonstrated a good normalized sensitivity of 533µV/(m/s)/mW for -1.29m/s to 1.29m/s nitrogen flow. Additionally, the sensor also displayed a good resolution of less than 3.2mm/s and a minimum detectable flow velocity (MDFV) of less than 1.6mm/s.
Thus, the proposed TMCF sensor can be used feasibly as a flow device for ultra-low fluid flow measurements in micro/nanofluidics.
More from AZoM: Semiconductors: Importance, Manufacture and How Gas Analysis Factors In
References and Further Readings
Fischer, A. C., Forsberg, F., Lapisa, M., Bleiker, S. J., Stemme, G., Roxhed, N., Niklaus, F. (2015). Integrating MEMS and ICs. Microsystems & Nanoengineering, 1(1), 1-16. https://doi.org/10.1038/micronano.2015.5
Qu, H. (2015). CMOS MEMS Fabrication Technologies and Devices. Micromachines, 7(1), 14. https://doi.org/10.3390/mi7010014
W. Xu., X. Wang., X. Zhao., Z. Ke., Y., -K. Lee. (2020). An Integrated CMOS Mems Gas Flow Sensor with Detection Limit Towards Micrometer Per Second. 2020 IEEE 33rd International Conference on Micro Electro Mechanical Systems (MEMS), 200-203. https://doi.org/10.1109/MEMS46641.2020.9056392.
Disclaimer: The views expressed here are those of the author expressed in their private capacity and do not necessarily represent the views of AZoM.com Limited T/A AZoNetwork the owner and operator of this website. This disclaimer forms part of the Terms and conditions of use of this website.