Today, a growing number of micro-manufactured actuators, sensors, and components vibrate at frequencies in the high MHz range. Some examples of such high-frequency vibrating systems are nano-electromechanical systems (NEMS), micro- electromechanical systems (MEMS), as well as ultrasound sensors and surface acoustic wave (SAW) filters, as used for imaging in medical applications, for example.
To test the functionality of these devices, to verify existing simulation models and to optimize the system design, their dynamic behavior needs to be measured. A laser vibrometer can be used to measure vibrations without making any contact, and these measurements are virtually non-invasive.
Until recently, Polytec vibrometers were limited to a highest measurement frequency of 24 MHz. The UHF-120 Ultra High Frequency Vibrometer now enables easy and reliable measurement of vibration characteristics of the systems below (Table 1) fo example up to a frequency of 1200 MHz.
Table 1. Typical frequency range of high-frequency components
Application |
Frequency range |
RF-MEMS |
1 MHz - >3 GHz |
SAW Filter |
10 MHz - >60 GHz |
NEMS |
50 MHz - >1 GHz |
HF Ultrasound Transducer |
1 MHz - >1 GHz |
Measurement Principle and Design of the UHF-120 Ultra High Frequency Vibrometer
The UHF-120’s operating principle is based on laser Doppler interferometry, the same as all other Polytec vibrometers. When a moving object is hit by the laser beam from the vibrometer, the phase and frequency of the light scattered back is shifted by the Doppler effect. This phase and frequency shift is measured interferometrically.
A heterodyne Mach Zehnder Interferometer is integrated into the UHF-120 sensor head, which includes the microscope optics (Figures 1 and 2). A beat signal occurs due to the interference of the returning Doppler shifted laser light with a reference beam. Beating is the interference of two coherent waves with a similar frequency.
If the object's position oscillates forwards and backwards ie. vibrates, a phase and frequency modulated signal is generated that includes all of the data on the object’s vibration. Using a commercial high frequency oscilloscope, this signal is sampled at a rate of up to 40 GigaSamples/second and then digitized. This digitized data is subsequently transferred to a PC via Ethernet where it is demodulated and analyzed using Polytec's software.
.jpg)
Figure 1. UHF-120 with sensor head (1) and controller (4).
.jpg)
Figure 2. Information flow in total UHF-120 system.
The UHF-120 system, shown in Figure 2, features the sensor head (1), oscilloscope (2), measurement computer (3), as well as the controller (4), which is the interface between the sensor head and measurement computer. If a scanned (surface) measurement is required, a motorized X/Y positioning stage can be used to expand the total system.
Measuring Ultrasound Sensors
The Fraunhöfer Institute for Biomedical Engineering (IBMT, St. Ingbert) developed both designs of ultrasound sensors to be measured. These sensors are used in medicine for measuring surfaces, cellular imaging, and abdominal imaging. The goal is to establish the 3D sound field dispersion in space by determining the out-of-plane vibration of tissue for example.
Operating Principle of Ultrasound Sensors
Both types of ultrasound sensor are capable of operating as transmitters and receivers of ultrasonic waves (ultrasound transducer). Based on the function and mode of operation of each piezo array, there is an impedance-matching layer (5 MHz) or a thin membrane (100 MHz Array) above the array.
When a voltage is applied to a defined field of the piezo array, it's possible to vary the amplitude as well as the phase of deflection of each piezo field. The piezo effect causes crystals to vibrate (Out-of-Plane), which is transmitted either to the thin membrane or a matching layer above it.
The adaptive layer or membrane then vibrates, generatering an ultrasound wave. The frequency and shape of this ultrasound wave can be changed by controlling the piezo field. If the correct phase and amplitude is selected for each array element, a focused ultrasonic wave with a variable point of focus can be generated with the low frequency sensor.
Measurements of Sensor 1 (Low-frequency Ultrasound Sensor)
This low frequency sensor is used for abdominal imaging. It has a surface area of about 10 x 10 mm2 with a vibration frequency of 5 MHz. A 5x objective was employed to measure, with the camera capturing a filed view of 1.42 x 1.1 mm2 .
To characterize the sensor across the entire surface, 30 individual fields (5 x 6) were scanned and determined continuously. 234 measurement points were defined in each area of interest (AOI).
During the scan, the phase and amplitude of vibration were recorded at every scanned location over a time span of 20 µs. A trigger signal to initiate the beginning of each separate measurement is also used as the phase reference. The data obtained from each scanned field were then combined and animated using Polytec's software.
Still images taken from the vibration animation can be observed in Figures 3 to 10. The overall vibration time is approximately 5 µs with a highest amplitude of ±40 nm (peak to peak). The vibration time time profile of a chosen measurement point is shown in Figure 11.
.jpg)
.jpg)
.jpg)
.jpg)
.jpg)
.jpg)
.jpg)
.jpg)
Figure 3 to 10. Vibration characteristics of the low-frequency ultrasonic sensor.
.jpg)
Figure 11. Amplitude time profile of a selected measurement point.
From the above images, it is apparent that ultrasonic surface waves can be generated in specific shapes with regulated excitation of a piezo array and a thin impedance matching layer above it. By observing the profile of the vibration in the first four individual images, it can be seen that the edge areasof the membrance followed by the middle areas are excited to vibrate.
The outer part of the ultrasonic wave starts earlier than the middle. As a result, the preferred type of spherical ultrasound wave is obtained, which after a certain distance fouses to a point.
Analysis of Data by the IBMT
The initial animations of measurement data obtained with Polytec's software already allowed the development team at IBMT to conclude that the membrane’s principle vibration behavior matches existing simulations. In addition, a comprehensive analysis of the measurement data made it possible to improve and correct the existing vibration model.
While studying the data, it should be noted that during the measurement the membrane was not operated in water as it would be in real life, but in air, without the damping created by the water.
The IBMT can now simulate the membrane vibration and the 3D propagation of the resulting ultrasonic wave more accurately using the updated simulation model. Laser Doppler vibrometry offers a considerably faster alternative to the more traditional measurement of 3D noise fields for evaluating transducer function.
Measurements of Sensor 2 (High-frequency ultrasound sensor)
This sensor is designed for making surface measurements as well as for cellular imaging. It operates at a vibration frequency of 105 MHz with a surface area of approximately 0.8 x 2.4 mm2.
When the high frequency ultrasound sensor is measured, a total of three adjacent areas were scanned and measured using the 10x objective. 405 sample points were assigned to each AOI.
In contrast to the low-frequency sensor, this sensor was covered with a 1 mm thick layer of water with a thin piece of cover glass placed on top. This helped make the measurement of the transducer more realistic.
Another difference from the low-frequency sensor, where a focused ultrasonic wave is generated by membrane vibration, the high frequency sensor can only have simultaneous excitation of the elements, and as a result, a sort of scan can be performed.
Based on which contacts points (Figure 12) are controlled on the sensor, various areas of the membrane are excited to vibrate. For instance, with a scanned ultrasonic measurement, different areas of the membrane can be made to vibrate in phase with each other, transmitting level sound waves at different positions. The sound waves created in this manner are reflected in the object being tested and are then detected by the ultrasound sensor.
.jpg)
Figure 12. Microscope image of the high-frequency ultrasonic sensor with a 1 mm thick layer of water and a cover glass.
The results, following excitation of four piezo fields in the middle of the ultrasound transducer, are shown in the following 9 pictures (Figures 13 – 21). It is clear from these images that the membrane’s surface vibration is created by the piezo array across a sharply defined area.
The settling of the membrane vibrating at about 105 MHz takes roughly 0.5 µs. The maximum amplitude of the membrane is a little more than 150 pm (Figure 13).
Based on the membrane’s deflection shape, it can be seen that due to strong attenuation by the water layer, only the area of the membrane excited by the piezos directly beneath it will begin to vibrate. Also the membrane clearly generates an almost flat, simultaneously focused and spatially sharp sound wave.
.jpg)
.jpg)
.jpg)
.jpg)
.jpg)
.jpg)
.jpg)
.jpg)
.jpg)
Figure 13 to 21.Vibration characteristics of the high-frequency ultrasound sensor.
Analysis of Data by the IBMT
Based on the out-of-plane vibration determined in water1, the IBMT can again spatially simulate the generated sound field using a model. The measurement in water acts as a realistic basis for the simulated sound field.
Conclusion
Vibration characteristics of high-frequency vibrating systems can be easily determined with high precision using the UFC-120 Vibrometer. In addition to high data accuracy, the short amount of time required is a major benefit of the UHF-120 Vibrometer which can be critical in the development process.
The time required to determine the ultrasonic field via surface measurement of the transducer was considerably reduced. As an alternative to measuring the 3D sound field using a hydrophones, the resulting sound field can be predicted with one or more scanned measurements and the subsequent simulation.
References
1 When using the high-frequency sensor, it was necessary to ensure that the sensor moved in water. The optical path, i.e. the path that the light takes, is calculated from the geometric path multiplied by the refractive index. Water with a refractive index of n = 1.33 has a larger refractive index than air (n = 1). Therefore the optical path of the light in water in a factor of 1.33 greater and the vibrometer measures a path that is a factor of 1.33 too large. To get the correct amplitude value, you must divide all of the measurement results by 1.33 which, takes a little effort using the PSV software together with the signal processor.
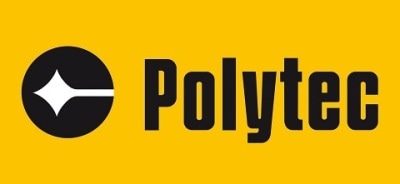
This information has been sourced, reviewed and adapted from materials provided by Polytec Ltd.
For more information on this source, please visit Polytec.