Additive Manufacturing (AM) involves the creation of three-dimensional objects, one superfine layer at a time, rendering it more costly than classical machining. However, only a small proportion of powder that is laid down in a build process is welded into a component. The rest is then unfused and consequently available for re-use. Contrastingly, if an object is classically created, it is usually necessary to remove material through milling and machining.
Powder properties govern machine parameters and should be considered first. Considering the unfused powder as contaminated and not adapted for reuse, the cost of AM will not be economical. Powder deterioration results in two phenomena: products chemistry modification and mechanical property change such as morphology and particle size distribution.
In the former, the main concern is to create solid structures that include pure alloys, so we need to avoid powder contamination such as oxides or nitrides. In the latter phenomenon, those parameters are related to flowability and spreadability. Therefore, any modifications in the powder characteristics could result in a product that cannot be evenly distributed.
Evidence in recent publications shows that the classical flowmeters are unable to give pertinent information about powder spreadability in powder-bed-based AM. Regarding raw materials (or powders) characterization, few relevant measurements methods are accessible in the market to answer this requirement. The stress state and the flow field of the powder should be analogous in the measurement cell and process. The existence of a compressive load is incompatible with the free surface flow used in AM devices in shear cell testers and classical rheometers.
GranuTools developed a workflow for AM powder characterization. Our main goal is to have one instrument per geometry that precisely mimics the process and this workflow was used to comprehend and trail the powder quality evolution after numerous printing processes. Several standard aluminum alloys (AlSi10Mg) were selected for different durations at diverse thermal stresses (from 100 to 200 °C).
It is possible to monitor thermal degradation by analyzing the powder’s ability to build up an electrical charge. The powders’ spreadability (GranuDrum instrument), packing dynamics (GranuPack instrument) and electrostatic behavior (GranuCharge instrument) were analyzed. Cohesion and packing dynamics measurements are appropriate in following powder quality.
A low cohesive Index is observed for a powder easy to spread, whereas a powder with a fastpacking dynamic will produce a mechanical piece with a lower porosity in contrast with a product harder to pack.
Material Methods
Selected Powders
Stocked in our lab for several months, three aluminum alloy powders (AlSi10Mg) and one stainless steel 316L sample with different particle size distribution were selected, known here as samples A, B and C. The sample properties might be different than other manufacturers. Sample grain size distribution was measured by laser diffraction analysis / ISO 13320.
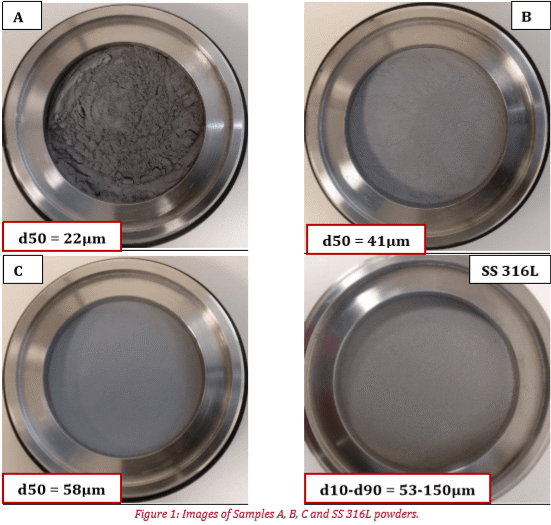
Methods
Since they govern machine parameters, powder properties should be the first consideration, and if we consider the unfused powder as contaminated and not adapted for re-use, the cost of AM will not be as economic as hoped. Due to this, three parameters will be investigated: powder spreadability, packing dynamic and electrostatics.
The spreadability involves the homogeneity and “smoothness” of the powder bed after the recoater operation. This is vital because a smooth surface is easier to print and will be investigated using the GranuDrum instrument with the cohesive index measurement.
Since pores represent weaknesses within the material, they can cause crack initiation. Packing dynamic is the second crucial parameter because a powder with fastpacking produces low porosity. This behavior is measured with the GranuPack with n1/2 values.
The presence of electric charges in a powder creates cohesive forces that lead to the formation of agglomerates. The GranuCharge measures the capability of powder to create electrostatic charges during a flow in contact with a selected material.
During processing, the GranuCharge can forecast the flowability deterioration, for example during the layer formation in AM. The resulting measurement is, therefore, highly sensitive to grain surface state (oxidation, contaminants and roughness). The aging of a recycling powder can then be accurately quantified (± 0.5 nC).
Spreadability Measurement: The GranuDrum
Based on the rotating drum principle, the GranuDrum instrument is a programmed powder flowability measurement method. A horizontal cylinder with transparent sidewalls is half-filled with a powder sample. The drum rotates around its axis at an angular velocity ranging from 2 rpm to 60 rpm meanwhile a CCD camera takes snapshots (30 to 100 images separated by 1s). The air/powder interface is identified in each snapshot with an edge detection algorithm.
The average interface position and fluctuations around this average position are calculated. For each rotating speed, the flowing angle (or ‘dynamic angle of repose’) αf is computed from the average interface position and the dynamic, cohesive index, σf, related to the cohesive forces between the grains, is analyzed from the interface fluctuations.
The flowing angle is influenced by a wide set of parameters: the friction, the shape and the cohesive forces between the grains (van der Waals, electrostatic and capillary). A cohesive powder leads to an intermitted flow while a non-cohesive powder leads to a regular flow. A low value of the flowing angle, αf, corresponds to a good flowability. A dynamic cohesive index close to zero corresponds to a non-cohesive powder, therefore when the powder cohesiveness upsurges, the cohesive index increases correspondingly.
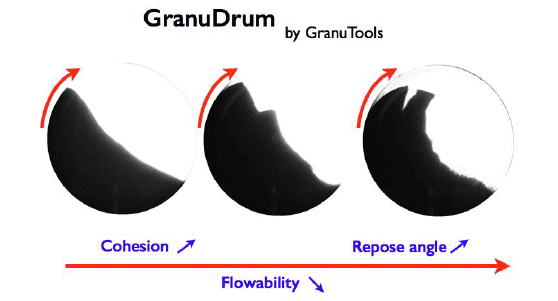
The GranuDrum allows the measurement of the first avalanche angle and the powder aeration during the flow as well as the measurement of both the cohesive index, σf, and the flowing angle, αf, as a function of the rotating velocity.
Packing Dynamics Measurements
Due to the simplicity and the rapidity of the measurement, GranuPack bulk density, tapped density and Hausner ratio measurement (also known as the “tap-tap test”) are very popular for powder characterization. The density and ability of a powder to increase its density are important parameters for storage, transportation, caking, etc. The suggested procedure is outlined in the pharmacopeia.
This simple test has three major drawbacks. The result of the measurement is dependent on the operator and the filling method affects the initial powder volume. Naked-eye volume measurements introduce strong result errors. With the simplicity of the experiment, we dismiss the compaction dynamics between the initial and final measurements.
The behavior of the powder presented to successive taps is analyzed with an automatized device. The Hausner ratio Hr, the initial density ρ(0) and the final density after n taps ρ(n) are measured precisely.
The tap number is commonly fixed at n=500. The GranuPack instrument is automated and improved tapped density measurement methods based on recent dynamic research.
Additional indexes can be used but are not presented here. The powder is put in a metallic tube with a rigorous automated initialization process. A dynamic parameter n1/2 and an extrapolation of the maximum density ρ(∞) are removed from compaction curves.
A light hollow cylinder is positioned on the top of the powder bed to keep the powder/air interface flat during compaction. The tube with the powder sample rose up to a fixed height of ΔZ and performs free falls at a height generally fixed to ΔZ = 1 mm or ΔZ = 3 mm, which is measured automatically after each tap. From the height, the volume, V, of the pile is calculated.
The density is the ratio between the mass m and the powder bed volume V. The powder mass m is known, and the density ρ is plotted after each tap.
The Hausner ratio Hr is connected to the compaction ratio and is analyzed by the equation Hr = ρ(500) / ρ(0), where ρ(0) is the initial bulk density and ρ(500) the tapped density computed obtained after 500 taps. The results are reproducible with a small quantity of powder (typically 35 ml) with the GranuPack method.
Electrostatic Measurements – The GranuCharge
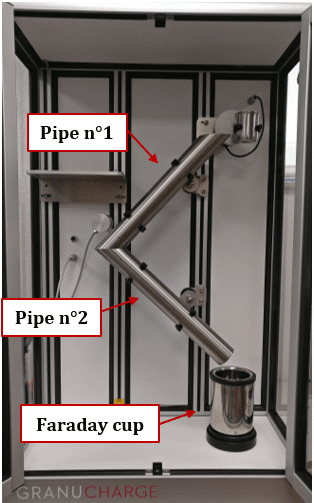
The characteristics of the powder and the nature of the material used to build the device are crucial parameters. Electrostatic charges are produced inside a powder during a flow and these charges are due to the triboelectric effect, which is a charge exchange at the contact between two solids.
During the flow of powder inside a device, the triboelectric effect occurs at the contact between the grains and at the contact between the grains and the device.
In contact with a selected material, the GranuCharge instrument automatically measures the number of electrostatic charges produced inside a powder during a flow. The powder sample flows inside a vibrating V-tube and fall in a Faraday cup connected to an electrometer that measures the charge acquired by the powder during the flow inside the V-tube. To acquire reproducible results, a rotating or vibrating device is used to feed the V-tube frequently.
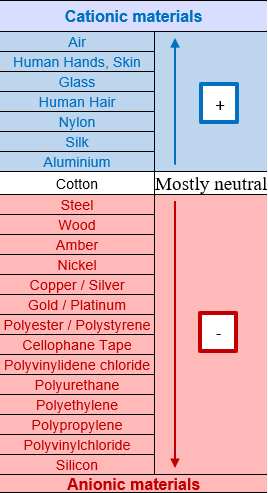
The triboelectric effect results in one object gaining electrons on its surface, and therefore becoming negatively charged, and another object losing electrons, consequently, becoming positively charged. Some materials have a greater tendency to gain electrons than others, in the same manner, that others tend to lose electrons easier.
Which material becomes negative and which becomes positive depends on the comparative tendencies of the materials involved to gain or lose electrons. To represent these trends, the triboelectric series was developed, as can be seen in Table 1. Listing materials with a tendency to charge positively and others with tendency to charge negatively, the middle of the table lists materials that do not show tendency to behave either way.
On the other hand, this table only gives information about material charging behavior tendency, which is why the GranuCharge was established to give exact numerical values about powders charging behavior.
Experimental Results
Several experiments were carried out to analyze thermal degradation. The samples were placed during one or two hours at 200 °C. Then the powders are immediately analyzed with the GranuDrum (“hot” designation). The powder is then placed in a container until it reaches ambient temperature, then analyzed using GranuDrum, GranuPack and GranuCharge (i.e. “cold”).
Virgin samples were analyzed with the GranuPack, GranuDrum, and GranuCharge at the same room humidity/temperature, i.e. 35.0 ± 1.5% RH and 21.0 ± 1.0 °C.
Spreadability
Experimental Protocol
The Cohesive Index calculates powder spreadability and is linked to the variations of the interface (powder/air) position, and it only represents the three contact forces (van der Waals, capillary and electrostatic). Before the experiment, air relative humidity (RH, %) and temperature (°C) are recorded. The powder is then poured inside the rotating drum cell, then the experiment is started.
Results
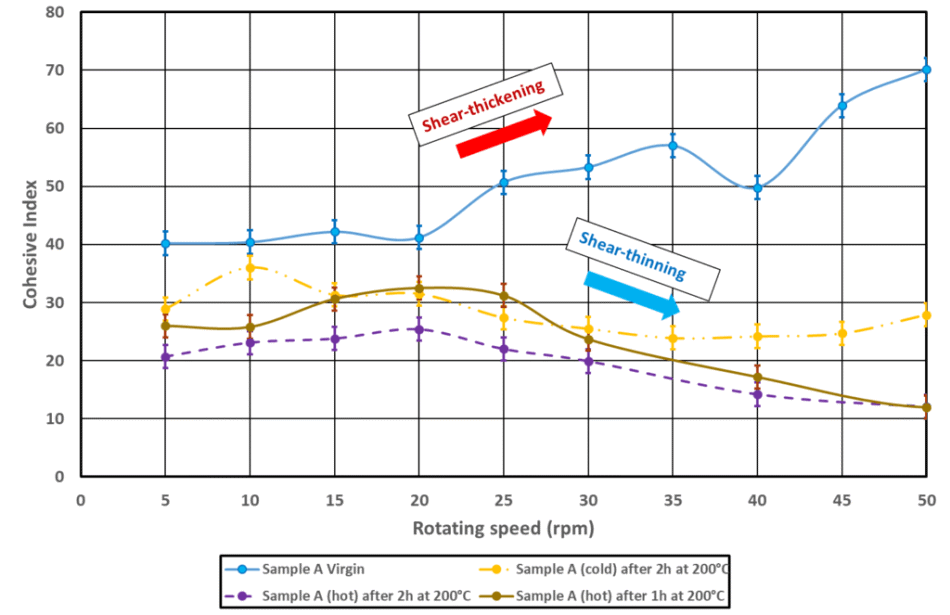
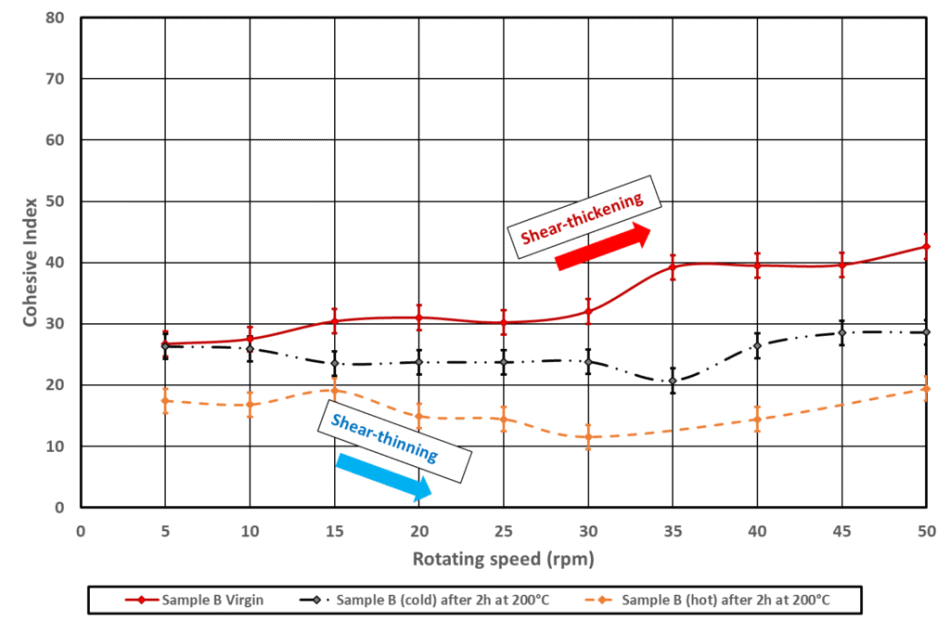
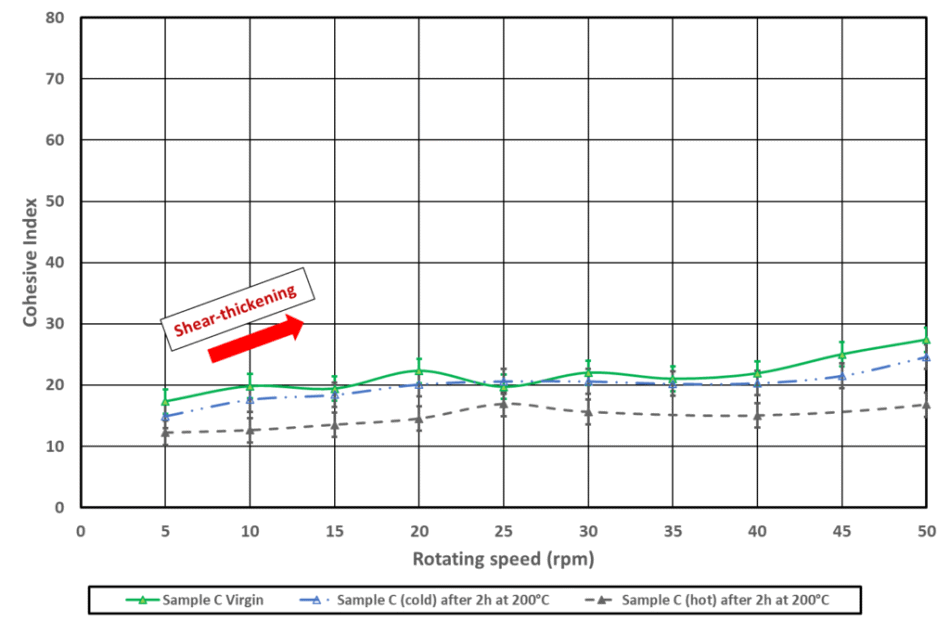
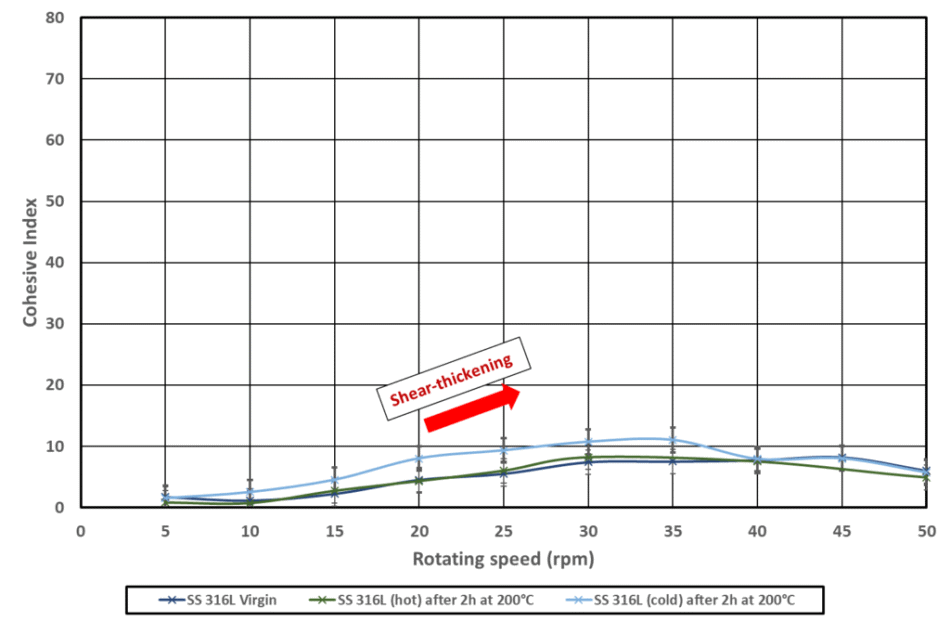
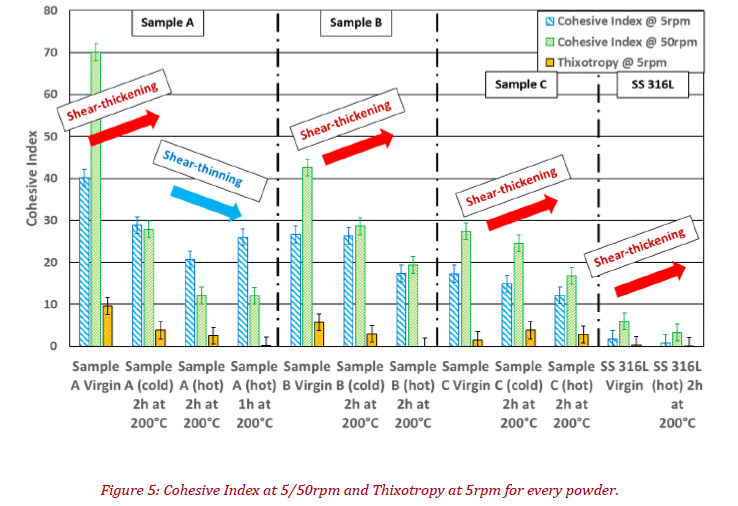
We conclude that those products are not sensitive to agglomeration when looking at the thixotrophy parameters. Interestingly, thermal stress changes the rheological behavior of the powder for samples A and B, going from shear-thickening to shear-thinning. Sample C and SS 316L, on the other hand, are not affected by temperature and only show a shear-thickening behavior. Each powder results in a better spreadability (i.e. a lower Cohesive Index) when heated up and after being cooled.
The temperature effect is also dependent on the particles’ specific area. The more material thermal conductivity there is, the more there is an effect on temperature (i.e. ??? 225°?=250?.?−1.?−1) and ??? 316? 225°?=19?.?−1.?−1) and the smaller the particles the more important the temperature influence. Working at high temperature is a succinct choice with aluminum alloy powders, due to their spreadability increase and even the cooled-down sample achieves improved spreadability in comparison with the virgin powders.
Packing Dynamics
Experimental Protocol
For each GranuPack experiment, the powder mass is recorded before each experiment and 500 taps were applied to the sample with a taps frequency of 1Hz and the measurement cell free-fall was 1 mm (∝ tap energy). By following the software instructions without user dependency, the sample is dispensed inside the measurement cell. Measurements were then repeated twice to evaluate the reproducibility and the average value and standard deviation were studied.
Once the GranuPack analysis is complete, the initial bulk density (ρ(0)), the final bulk density (at a number of taps, n = 500 ; i.e. ρ(500)), the Hausner ratio/Carr index (Hr/Cr), and two recorded parameters linked to the compaction dynamics (n1/2 and τ). The optimal density ρ(∞) is also displayed (see Appendix 1). The following table reorganizes the experimental data.
Results
Figures 6 and 7 symbolize the full compaction curves (bulk densities versus number of tap) and n1/2 parameter/Hausner ratio. Error bars calculated using the average value have been displayed on each curve and standard deviation was calculated with the repeatability tests.
The stainless steel 316L product is the heaviest one (ρ(0) = 4.554 g/ml). Regarding the tapped densities, SS 316L is still the heaviest powder (ρ(n) = 5.044 g/ml), sample A comes in second position (ρ(n) = 1.668 g/ml) followed by sample B (ρ(n) = 1.645 g/ml). Sample C has the lowest (ρ(n) = 1.581 g/ml). In terms of bulk densities for the virgin powders, we see that sample A is the lightest and B and C accomplish approximately the same values if error bars are considered (1.380 g/ml).
If the powder is heated, its Hausner ratio decreases and this only happens for sample B, C and SS 316L. For sample A, because of the error bar size, it is impossible to accomplish. For the n1/2, parameter trends are more complicated to underline. For sample A and SS 316L, n1/2 decreases after 2 hours at 200 °C, while it increases for powders B and C following thermal stress.
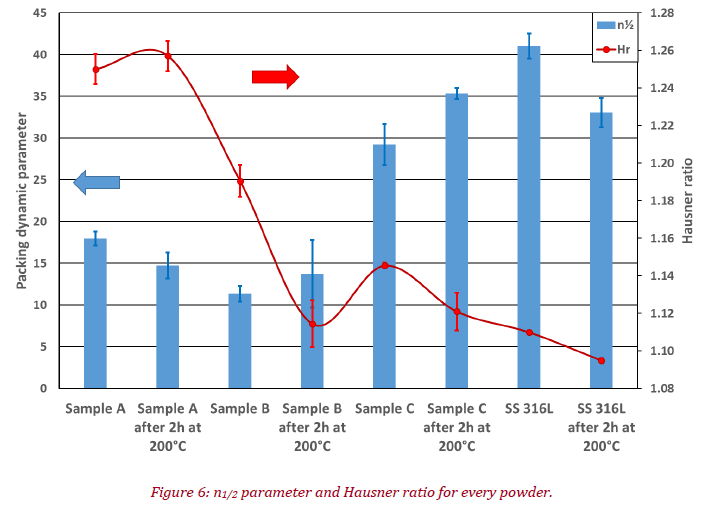
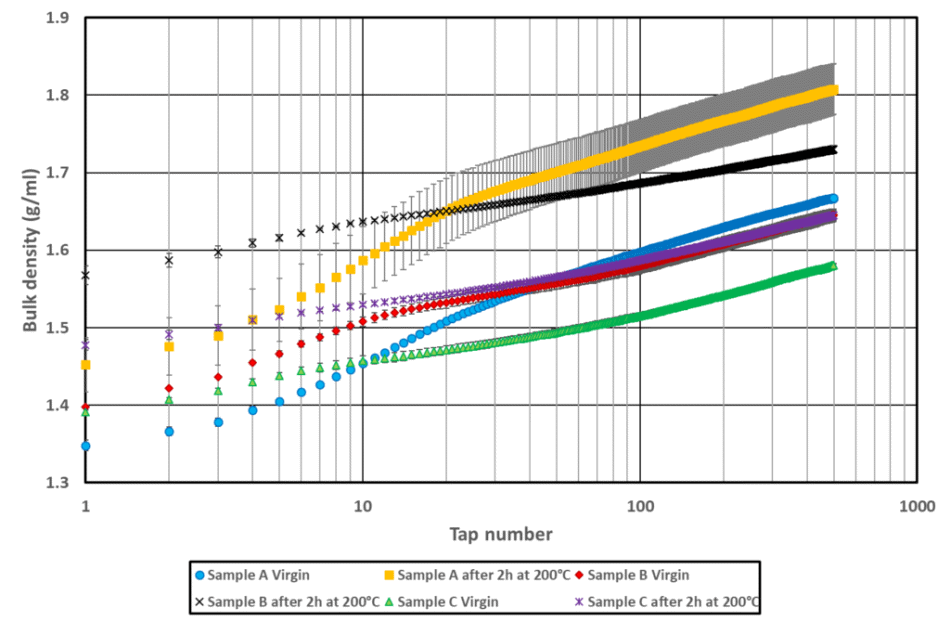
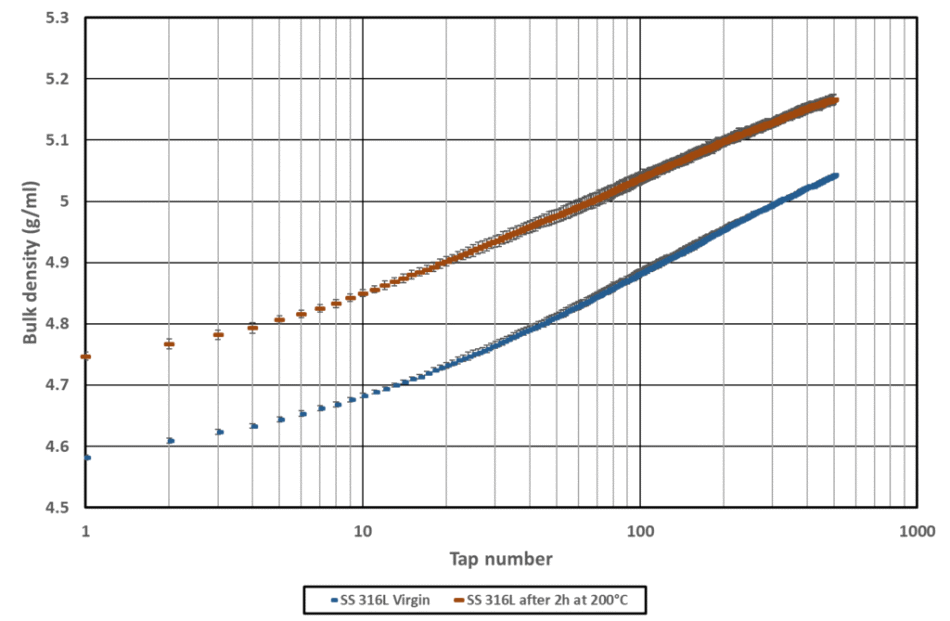
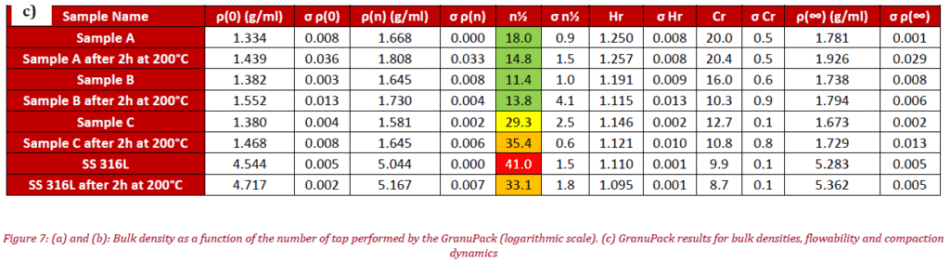
Electrostatics
Experimental Protocol
For each GranuCharge experiment, the vibrating feeder was used (see Figure 8). Stainless-steel 316L pipes were selected. Measurement have been repeated three times for evaluating reproducibility. The product quality used for each measure was around 40 ml and the powder was not recycled after measurement.
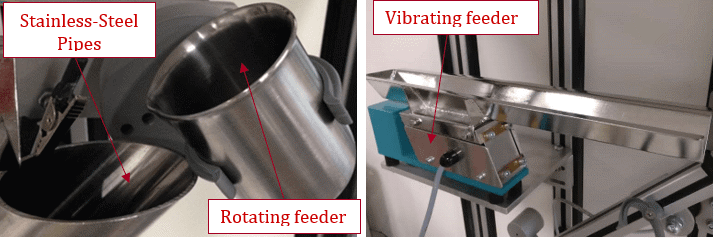
Prior to the experiment, powder mass (mp, in g), air relative humidity (RH, %) and temperature (°C) are recorded. At the start of the test, the primary powder charge density (q0, in μC/kg) is measured by introducing powder inside the Faraday cup. At the end, powder mass is recorded and final charge density is computed at the end of experiment (qf, μC/kg) and Δq (Δq = qf – q0).
Results
GranuCharge raw data is shown in table 2 and figure 9 (σ is the standard deviation calculated with the repeatability tests) and the results displayed as a histogram (only q0 and Δq are presented). SS 316L has the lowest amount of initial charges; this could be because this product has the highest PSD. When it comes to the virgin aluminum alloy powder’s initial charges, it is impossible to conclude due to error bars size.
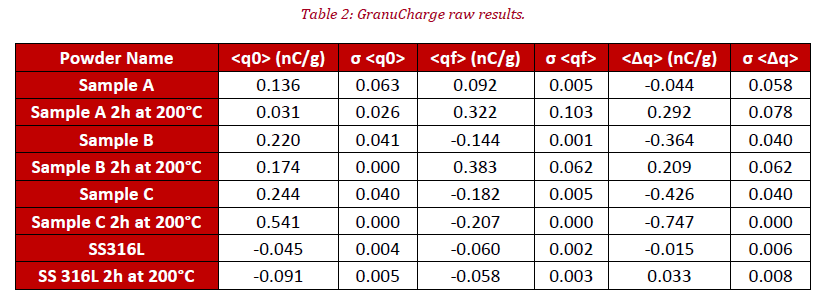
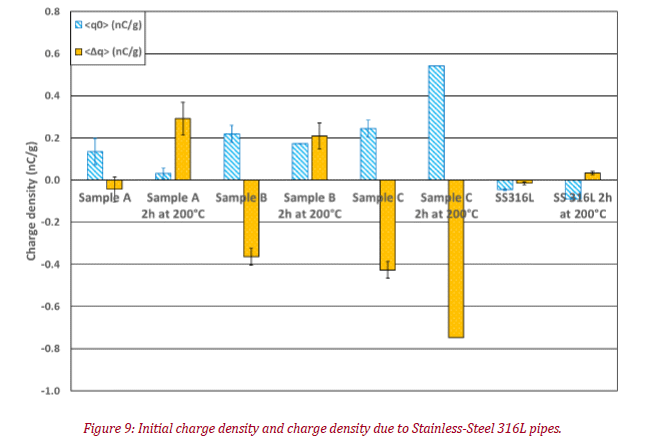
After a flow in contact with stainless steel 316L pipes, sample A acquires a lowest amount of charges, in comparison with powder B and C which emphasize similar trends and if a SS 316L powder is rubbed against a SS 316L, a charge density close to 0 is detected (see the triboelectric series). Product B is still more charged than A. For sample C, the trend is conserved (positive initial charge and final charge after a flow), but the number of charges is increased following thermal degradation.
Powder behavior becomes quite fascinating after a thermal stress of 200 °C for 2 hours. In sample A and B, initial charges reduce and the final charge converts from negative to positive. SS 316L powder has the highest amount of initial charges, and its charge density variation changes to positive but stays low (i.e. 0.033 nC/g).
Conclusions
We have investigated the effect of thermal degradation on the combined behavior of aluminum alloys (AlSi10Mg) and a stainless steel 316L powder, meanwhile, virgin powders were analyzed under ambient air after 2 hours at 200 °C.
Working with powder at a high temperature improves the product spreadability, and this effect seems to be more important for powders with high specific area and for a material with high thermal conductivity. The GranuDrum was used for the spreadability assessment, the GranuPack for packing dynamic analysis and the GranuCharge to analyze powder triboelectricity in contact with stainless steel 316L pipes.
These results were established by the GranuPack, where an improvement of the Hausner ratio is shown for every powder (except sample A, due to error bars size) after the thermal stress process. Looking at the packing parameter (n1/2), no well-defined trends were attained, since some products highlight the enhancement in terms of packing speed, and others have a contrasting effect (such as sample B and C).
Since their charge sign was changed, some products were highly impacted by the thermal degradation process and the variances between the aluminum alloys and stainless steel 316L powder was also easy to emphasize since the SS 316L sample was not highly charged after flow in contact with the SS 316L pipes.
References and Further Reading
- Cascade of granular flows for characterizing segregation, G. Lumay, F. Boschin, R. Cloots, N. Vandewalle, Powder Technology 234, 32-36 (2013).
- Combined effect of moisture and electrostatic charges on powder flow, A. Rescaglio, J. Schockmel, N. Vandewalle and G. Lumay, EPJ Web of Conferences 140, 13009 (2017).
- Compaction dynamics of a magnetized powder, G. Lumay, S. Dorbolo and N. Vandewalle, Physical Review E 80, 041302 (2009).
- Compaction of anisotropic granular materials: Experiments and simulations, G. Lumay and N. Vandewalle, Physical Review E 70, 051314 (2004).
- Compaction Dynamics of Wet Granular Assemblies, J. E. Fiscina, G. Lumay, F. Ludewig and N. Vandewalle, Physical Review Letters 105, 048001 (2010).
- Effect of an electric field on an intermittent granular flow, E. Mersch, G. Lumay, F. Boschini, and N. Vandewalle, Physical Review E 81, 041309 (2010).
- Effect of relative air humidity on the flowability of lactose powders, G. Lumay, K. Traina, F. Boschini, V. Delaval, A. Rescaglio, R. Cloots and N. Vandewalle, Journal of Drug Delivery Science and Technology 35, 207-212 (2016).
- Experimental Study of Granular Compaction Dynamics at Different Scales: Grain Mobility, Hexagonal Domains, and Packing Fraction, G. Lumay and N. Vandewalle, Physical Review Letters 95, 028002 (2005).
- Flow abilities of powders and granular materials evidenced from dynamical tap density measurement, K. Traina, R. Cloots, S. Bontempi, G. Lumay, N. Vandewalle and F. Boschini, Powder Technology, 235, 842-852 (2013).
- Flow of magnetized grains in a rotating drum, G. Lumay and N. Vandewalle, Physical Review E 82, 040301(R) (2010).
- How tribo-electric charges modify powder flowability, A. Rescaglio, J. Schockmel, F. Francqui, N. Vandewalle, and G. Lumay, Annual Transactions of The Nordic Rheology Society 25, 17-21 (2016).
- Influence of cohesives forces on the macroscopic properties of granular assemblies, G. Lumay, J. Fiscina, F. Ludewig and N. Vandewalle, AIP Conference Proceedings 1542, 995 (2013).
- Linking compaction dynamics to the flow properties of powders, G. Lumay, N. Vandewalle, C. Bodson, L. Delattre and O. Gerasimov, Applied Physics Letters 89, 093505 (2006).
- Linking flowability and granulometry of lactose powders, F. Boschini, V. Delaval, K. Traina, N. Vandewalle, and G. Lumay, International Journal of Pharmaceutics 494, 312–320 (2015).
- Measuring the flowing properties of powders and grains, G. Lumay, F. Boschini, K. Traina, S. Bontempi, J.-C. Remy, R. Cloots, and N. Vandewalle, Powder Technology 224, 19-27 (2012).
- Motion of carbon nanotubes in a rotating drum: The dynamic angle of repose and a bed behavior diagram, S. L. Pirard, G. Lumay, N. Vandewalle, J-P. Pirard, Chemical Engineering Journal 146, 143-147 (2009).
- Mullite coatings on ceramic substrates: Stabilisation of Al2O3–SiO2 suspensions for spray drying of composite granules suitable for reactive plasma spraying, A. Schrijnemakers, S. André, G. Lumay, N. Vandewalle, F. Boschini, R. Cloots and B. Vertruyen, Journal of the European Ceramic Society 29, 2169–2175 (2009).
- Rheological behavior of β-Ti and NiTi powders produced by atomization for SLM production of open porous orthopedic implants, G. Yablokova, M. Speirs, J. Van Humbeeck, J.-P. Kruth, J. Schrooten, R. Cloots, F. Boschini, G. Lumay, J. Luyten, Powder Technology 283, 199–209 (2015).
- The flow rate of granular materials through an orifice, C. Mankoc, A. Janda, R. Arévalo, J. M. Pastor, I. Zuriguel, A. Garcimartín and D. Maza, Granular Matter 9, p407–414 (2007).
- The influence of grain shape, friction and cohesion on granular compaction dynamics, N. Vandewalle, G. Lumay, O. Gerasimov and F. Ludewig, The European Physical Journal E (2007).
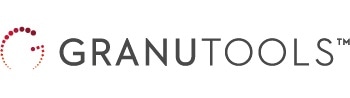
This information has been sourced, reviewed and adapted from materials provided by Granutools.
For more information on this source, please visit Granutools.