In this interview, we talk to Alex de Marco, an Associate Professor at Monash University, about the correlative use of light and electron microscopy in the study of biological samples, as well as the use of multi-ion plasma focused ion beam (plasma FIB) technology in his lab.
Tell us a bit about your background with electron microscopy and other microscopy techniques.
I got involved with electron microscopy during my Ph.D. studies at the EMBL (European Molecular Biology Laboratory). This was before the so-called “cryo-EM resolution revolution,” so we were still developing methods for sub-tomogram averaging and structural biology on cryo-electron microscopes.
When I finished my Ph.D., I left academia for a while and started to work for FEI, which is now part of Thermo Fisher Scientific. I was there for roughly four years, initially in R&D, and then in product management, and was responsible for anything that had to do with volume imaging and correlative microscopy.
That’s basically where I started to work on both electron and light microscopy together, and where I first became interested in performing in situ structural biology, which is now one of the major focuses of my lab.
Can you tell us a bit more about your group's work?
Basically, what we do is method development, as well as a bit of hardware development. We’re trying to facilitate imaging, with the ultimate goal of simplifying the process of in situ structural biology.
One thing that I helped to do at the end of my Ph.D. was simplifying some processes surrounding electron microscopy. At the time, EM was very difficult, because all the current automation was still not in place. It was, for all intents and purposes, a field. And what I find interesting is that, as soon as you facilitate enough of the operations in a field, it becomes a tool. And then research can be done using the tool.
So, what we're trying to do is facilitate the application of various correlative microscopy techniques, so that you don’t need to have 10 years of experience to perform these types of experiments.
Our second, parallel focus is microfabrication. In particular, we are working on novel optical elements, specifically in the micro-optics space, with potential applications spanning from imaging to lithography. However, at the moment, we are primarily focusing on the physical optics side, rather than on these applications.
Your group has recently developed the photon ion-electron microscope (PIE-scope), an integrated cryo-FIB, and a light microscope. Can you tell us what motivated the development of this instrument?
While performing cryo correlative microscopy there are always two major problems. First, it doesn’t matter how good your light microscope is, and it doesn’t matter how much you try to simplify transfers between instruments, there will always be some contamination and sample loss.
The second problem is the time. If you think about it, the biggest expense in any lab is salary. When you have someone who’s highly skilled, and they’re just spending their time browsing through a sample and waiting in front of a screen, you’re not really making the best use of your resources.
So, the solution to both problems is to have processes that are as automated as possible. To do that, you need to make sure that the different components of the workflow can talk to each other. For us, the simplest way to do that was to integrate everything. And it worked out really well because we were able to come up with a solution where we didn't have to compromise on anything.
With the PIE-scope, we are able to perform imaging at the highest possible resolution without using immersion. So, the quality is equivalent to any stand-alone cryo light microscope, without any impact on FIB or scanning electron microscopy (SEM) performance. Practically speaking, we ended up making something which truly just simplifies people's life.
What can you do with the PIE-scope that wasn't possible before?
Well, we can screen many grids, immediately, before a sample is prepared. Previously, you could only really work with perfect samples, but now, it no longer matters if one of your samples has few ideal regions, or if another one has many more. Once the sample is in, you just prepare the lamella with a FIB, even if you’ve only got a single good spot. The screening can happen automatically because everything is inside an enclosed environment which is motorized and fully computer-controlled.
Basically, the instrument saves time. It’s also cheaper than buying separate microscopes, all the cost of the enclosure/base and dedicated electronics is saved, and you do not need any dedicated lab space for it.
Grid preparation is definitely still a hurdle for scientists working with cryo-EM, so I can imagine how removing even some of the people’s frustrations can have a huge benefit.
Yeah, and there are other advantages. For example, some of the problems in correlative microscopy arise when you change modalities because you never know what the distortions between different instruments will be. In the case of the PIE-scope, everything is fixed, and once you've calibrated, you don't really have to do anything. You just basically line up two images.
You’re one of the first scientists to really explore plasma FIB for life science applications. What were some of the challenges you faced when you first began, and how has plasma FIB been integrated into your lab’s work?
I really started exploring plasma FIB applications at FEI. At the time, I was interested in speeding up sample preparation because, as a researcher, you want to analyze data. You don't want to sit there and watch the microscope do something for 15 minutes, and then start again, and so on, because that eats away your day. I thought the use of plasma FIB might be a potential route forward, and the R&D team, which is fantastic, showed us a multi-ion source [now part of the Helios Hydra DualBeam].
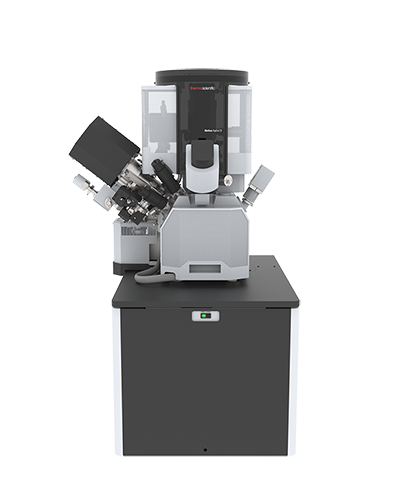
Helios Hydra DualBeam
This was interesting because xenon, the typical ion species for plasma FIB, is optimized for semiconductors, so for some samples, it doesn’t perform well and is very difficult to use. In these cases, it’s great to have the opportunity to play with three other ion species [oxygen, nitrogen, and argon] with the push of a button. It’s like having four different microscopes in one. So, we started to explore what the different ion sources could do for biological samples.
As it turns out, however, this was an even greater opportunity than we initially thought. With plasma FIB, sample preparation can be done very quickly, and the beam damage can be very contained. So, the fabrication of relatively large patterns is extremely fast. And that's where we started to work on micro-optics, which was not a focus of my lab until I had the Helios Hydra.
What we do now simulate thousands of different lenses. We can make them in 10 minutes or less, and then test to see if they are good or not. This has completely changed the way we perform research because, in physical optics, there have been a lot of articles, and a lot of theoretical research, that came out in the past 15-20 years. And everything was theoretical because there was no easy way to actually fabricate the lenses.
Now, we just make them and check whether the theoretical work is actually correct. In the future, this will bring a lot of advantages to beam shaping and the construction of very complex beams.
On the biological side of things, one of the major users of the machine is for volume acquisition, what’s called FIB-SEM tomography. The idea is you polish a face with a FIB, and then you image it. And then you polish again, and you iterate this process as many times as you need to produce a 3D reconstruction of the sample being studied. Here we found that the oxygen plasma beam produces great results because it forms a very flat surface quickly (up to 2x faster than gallium FIB) and with minimal artifacts.
Notably, while imaging was twice as fast, the area you could prepare scaled linearly, it didn’t scale up exponentially. So, we could now prepare an area 8-10x larger [compared to gallium FIB] in a reasonable amount of time. Of course, this leads to a new problem, since the original aim was to speed up data collection, and what we did instead is just collect more data. So, we really didn’t accomplish our goal, but I suppose that’s just human nature.
But generally speaking, what's happening is that we’re now able to examine entire regions of tissue and see how events occur in a much larger environment. For us, that's been really revolutionary. When we do prepare lamella, we find that it takes about 30% less time compared to conventional gallium FIB. And the lamella can be extremely thin, so lamella quality also seems to be higher.
We’re also producing better quality TEM micrographs; we don't quite know why this is happening but understanding this process would be extremely useful. Ultimately though, the real advantage is, again, time, and the fact that we can mill and prepare very large samples.
For example, multiple labs have recently started doing cryo lift-out sample preparation, where you prepare a lamella directly from the sample rather than from a single isolated cell. To do this, you essentially have to clear out tissue surrounding the region that you want to lift out, which requires very large trenches. With the plasma FIB, we can do this in a matter of minutes as opposed to gallium, which can easily take hours.
Is this all done with the oxygen PFIB? Or are you coarse milling with one ion, then polishing with another, etc.?
Typically, the coarse milling happens with oxygen because it appeared to be the most efficient in clearing the bulk. Likely there is some chemical effect occurring in addition to sputtering because we’re seeing low redeposition of carbon-based material.
However, what we did find is that polishing with oxygen is not quite ideal, and causes more damage than we would like. Here, we started to work with argon and xenon, since we can easily switch. And at the moment, we're still trying to understand the benefits are of using one over the other for polishing.
What do you see as the future of FIB for the life sciences, as well as the field of cryo-electron microscopy in general?
Personally, I hope that the field stops being a field, and instead, becomes a tool. Maybe that’s not the hope of everyone, but I would like it to be similar to how we currently view fluorescence microscopy. The action of imaging your sample with cryo-EM should not be the major focus of your research; it should just be the tool that gives you the data. So ideally, everything should become streamlined, even for in situ subcellular biology.
For example, things are becoming more and more automated in single particle analysis (SPA). For some simpler proteins and complexes, there are virtually no manual interactions needed from the moment you've set up data collection to when you actually have a structure. This doesn't work for every protein, but it works for enough cases that more labs are actually getting into SPA, especially those focusing more on the biology or pharmacology side of things versus pure structural biology. For them, this is already more of a tool. It gives them the information they want and then they walk away, and they perform some other experiments. And ideally, this is what I would like to see more of for in situ structural biology as well.
Getting there is not quite as straightforward, however. With current advances in FIB and TEM automation, we can probably look at 4-5 lamella an hour, resulting in 4-5 corresponding tomograms. The data is there, but then we reach a significant bottleneck.
When you look at your tomogram, you can recognize ribosomes, vesicles, even membranes, and major organelles. But practically, the question that pretty much everybody will ask at some point is, “what’s that blob?” Because in many cases, what you have in front of you is basically a blob that you cannot identify. You can only guess at what it could be.
We need to find ways to label these tomograms and data in a meaningful and efficient manner. There has already been some effort in this regard, for example, template matching, which can be very efficient. But generally, we're still at the point where we’re asking, “what’s that blob?” when we look at the electron density inside a cell. Answering this question reliably is probably the next big challenge.
It becomes a question of interpretation, not just image quality.
That’s right, and all the answers are not going to come from electron tomography only. You will need a priori information which you acquired with crystallography, or other methods, to actually understand what you see in your tomograms.
So, the road to in situ structural biology as a tool seems quite long, but these are exciting times, because we’re no longer limited by computational power, and more and more data is becoming available. The number of protein structures being found per year just keeps increasing, and we’ll likely have enough structures soon to use them to interpret tomograms.
About Alex de Marco
I obtained my Ph.D. at EMBL working in the group of Dr. John Briggs. Here, I contributed to the development of image processing for high-resolution cryo-electron tomography and sub-tomogram averaging. After my Ph.D. I moved to FEI Company (now Thermo Fisher Scientific) where I have been a product manager for FIB/SEM microscopes and correlative microscopy. At the end of 2015, I established my laboratory at Monash University. Here, my research focuses on methods and hardware development for focussed ion beam microscopy, electron microscopy, and correlative FIB/SEM microscopy.
Disclaimer: The views expressed here are those of the interviewee and do not necessarily represent the views of AZoM.com Limited (T/A) AZoNetwork, the owner and operator of this website. This disclaimer forms part of the Terms and Conditions of use of this website.