AZoM spoke with Dr. Qing Tu about his research to understand the durability of 2D hybrid organic-inorganic materials. This development could have far-reaching real-world applications in flexible electronics and optoelectronics in the near future.
Please could you introduce yourself to our readers and tell us about your background in materials science?
My name is Qing Tu, and I'm an assistant professor in the Department of Materials Science Engineering at Texas A&M University. Previously, I completed my Ph.D. at Duke University, in the Mechanical Engineering and Materials Science Department. After completing my Ph.D. I was a postdoctoral researcher at Northwestern, again in the Materials Science and Engineering Department. My main focus is on trying to understand the surface and interfacial properties of advanced functional materials for energy and electronic applications and trying to harness the new understandings to engineer those surfaces and interfaces for improved performance.
What are 2D hybrid materials, and what fields are they most relevant to?
We call them 2D hybrid organic-inorganic perovskites, which refer to a family of solution-processed, 2D semiconductor materials with both organic and inorganic bondings at the molecular level. These materials are derived from their 3D parent crystals, three-dimensional hybrid organic-inorganic perovskites. Initially, these materials caught people's attention because they have huge potential for use in optoelectronic applications like solar cells. The chart from the National Renewable Energy Lab in the United States shows that the solar cells made of these hybrid organic-inorganic perovskites deliver extremely efficient performance. Then, later on, it was discovered that these materials are really good semiconductor materials with very low production costs because of the scalable solution processing methods, and their optical and electronic properties manifest high tolerance for defects.
What is the significance of high tolerance in a hybrid organic-inorganic material showing defects?
It means even though you can produce it in a solution with a lot of defects, the performance remains acceptable for a wide range of applications. This feature triggered significant interest in the fundamentals of these properties. Why are they showing these unique properties, and can we use 2D hybrids for other applications?
Due to the fact a 2D hybrid material is a semiconductor, indeed the application field is greatly expanded. For instance, it can be used in LEDs, flexible electronics, radiation detection, memory transistors; literally the whole spectrum of semiconducting applications.
Would you say it’s the potential for wide application use that led you to study 2D hybrid organic-inorganic materials?
Yes, I first became aware of this field when I was a graduate student in 2015. I noticed that there were more and more people talking about perovskites, and then I started to turn my attention toward this field. My Ph.D. is on 2D materials, specifically graphene and their surface and interfacial properties. Then towards the end, I started to look into if and how we can engineer the function properties of 2D perovskites through the chemical compositions. We started to collaborate with chemists and discovered that the organic components could tailor the crystal symmetry of these 2D structured crystals and make some of them become piezoelectric, which further expands their application field. When I moved to Northwestern, I started to look more into the structure-property relationship of this family of materials, particularly on how different chemistry and different components had an effect on their mechanical behaviors.
How does your research differ from previous studies on the topic?
Unlike the mainstream research on 2D hybrid organic-inorganic perovskites, where people mostly focus on the optoelectronic behavior of these materials, I tend to focus more on the mechanical behavior of these materials. This, I think, is good motivation for investigating any real-world applications because of the universal presence of mechanical strain and the critical influence of mechanical strain on the reliability and performance of these materials and their devices.
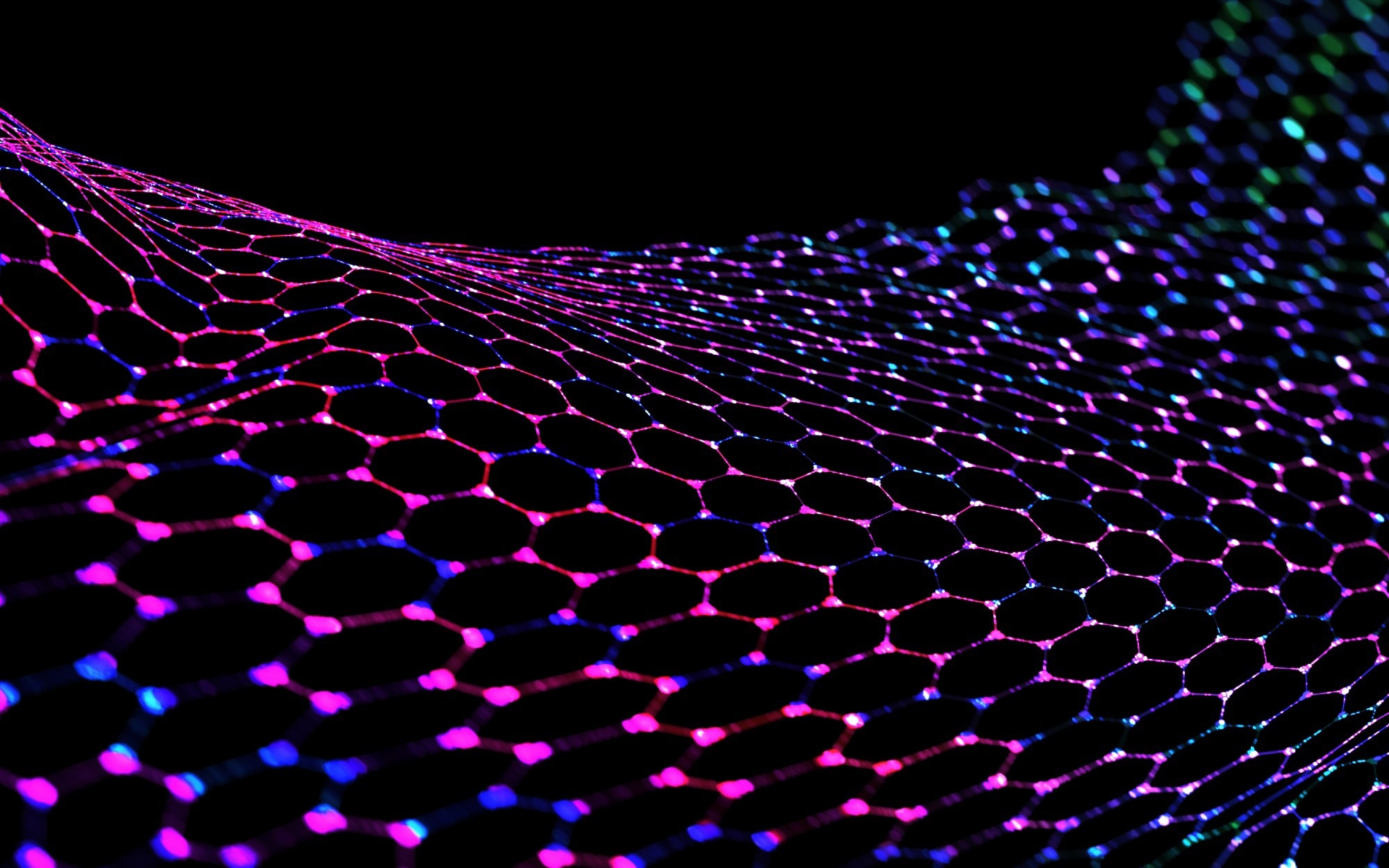
Image Credit: Marco de Benedictis/Shutterstock.com
Recently, we have been studying fatigue behavior. Materials have mechanical strengths. When the mechanical stress reaches the strength of the material, the material will fracture. However, in reality, the loading conditions that the material experiences during device application are usually subcritical. That means the stress field is actually less, lower than the critical strength. And this loading can also be cyclic. Over a long time, this cyclic subcritical mechanical stress can still drive the mechanical failure of the materials. One way to understand this is if you take a metal wire. When you try to stretch the metal wire, it's usually very difficult to break it - we cannot do that with our hands. Yet, if you bend it many, many times, back and forth, although each time you're not breaking it, the wire will eventually break. That's called a fatigue behavior or a fatigue phenomenon.
Prior to this study, we looked into how different chemistry and structure in the material affected its properties. We call it the quasi-static mechanic property of these materials. But the fatigue behavior of these materials remains unknown. Yet for practical applications, you do have these materials suffering from this subcritical oscillative stress coming from various sources like temperature fluctuation, flexible electronic deformation, and also sometimes from the piezoelectric, or maybe from the electro-mechanical coupling behavior arising from the material itself.
Many others realized that this was a critical problem. They studied how these perovskite-based solar cells, or flexible electronics, will fail under multiple cycles of bending. Typically people monitor the efficiency as a function of bending cycles at different levels of bending radius, but in all those studies, the stress or strain is not quantitative; it's rather a qualitative metric relative to what radius you are bending to. Furthermore, due to other complexities of devices, many factors can contribute to the observed fatigue failure in these studies.
It can be failure of the electrodes, failure of the interface, or failure of the other interfacing materials such as charge transporting materials or other components inside the devices. Hence these prior studies can hardly provide quantitative fundamental insights into the fatigue behavior of 2D perovskites. This is why we want to focus on the fundamental properties of the 2D perovskites themselves and to understand how this subcritical cyclic loading will lead to the failure of the material and what that failure behavior will be like.
On the other hand, previous studies do consider the fatigue failure of 2D materials like pure inorganic 2D materials such as graphene, MoS2, and boron nitride, but this is the first time 2D materials with hybrid features with both organic and inorganic hybrid components in the crystal structure have been the primary focus.
Throughout your research, you have looked at how these 2D inorganic-organic perovskites can survive over 1 billion cycles. What are the implications of that when it comes to these real-world applications?
I think that it is good news for people who want to use perovskite in real-world applications because, at least fundamentally, we show the material itself can survive long cycles. In typical engineering applications, we are talking about a fatigue lifetime, about 10-to-the-five or 10-to-the-six cycles, and this one you can survive 1 billion cycles under typical device operating conditions.
Of course, there are some conditions you still have to take into account. For instance, you cannot load it too high, but when subjected to similar conditions, the hybrid perovskites outperform typical polymers, particularly those widely used as substrates for flexible electronics.
How is it possible that these materials are so enduring?
This was something new to us as well when we started because the 3D parent crystals of these 2D hybrid perovskites are brittle. It's not expected that these 2D hybrid perovskites will survive such a tremendous number of study cycles. When we first saw the results, we were really excited.
We believe it's probably due to some unexpected plastic deformation mechanism inside this 2D form of hybrid organic-inorganic perovskites because, based on the failure behavior, we noticed that when you have relatively mild loading conditions, the material tends to fail plastically.
That means the damage generated will be contained, avoiding any catastrophic cracks that propagate all the way through the material. This feature provides a mechanism for the material to constraint the damage locally and thus extend the lifetime of the material, which is in stark contrast to a 3D perovskite (the 3D parent) which is an ionic, brittle crystal. Once you have a failure in the 3D parent material, the crack will just propagate all the way through.
What makes 2D hybrids so well-suited to flexible electronics, and is that an application area that you can grow into something much larger?
There is a significant amount of effort being put into pursuing their suitability for application in flexible electronics. Firstly, this is because it's solution processable, so it's scalable and highly compatible with many scalable industrial processing methods. Secondly, it is low cost, and at the same time, the performance is still quite good because of the high defect tolerance of the optoelectronic and semiconductor properties of these materials.
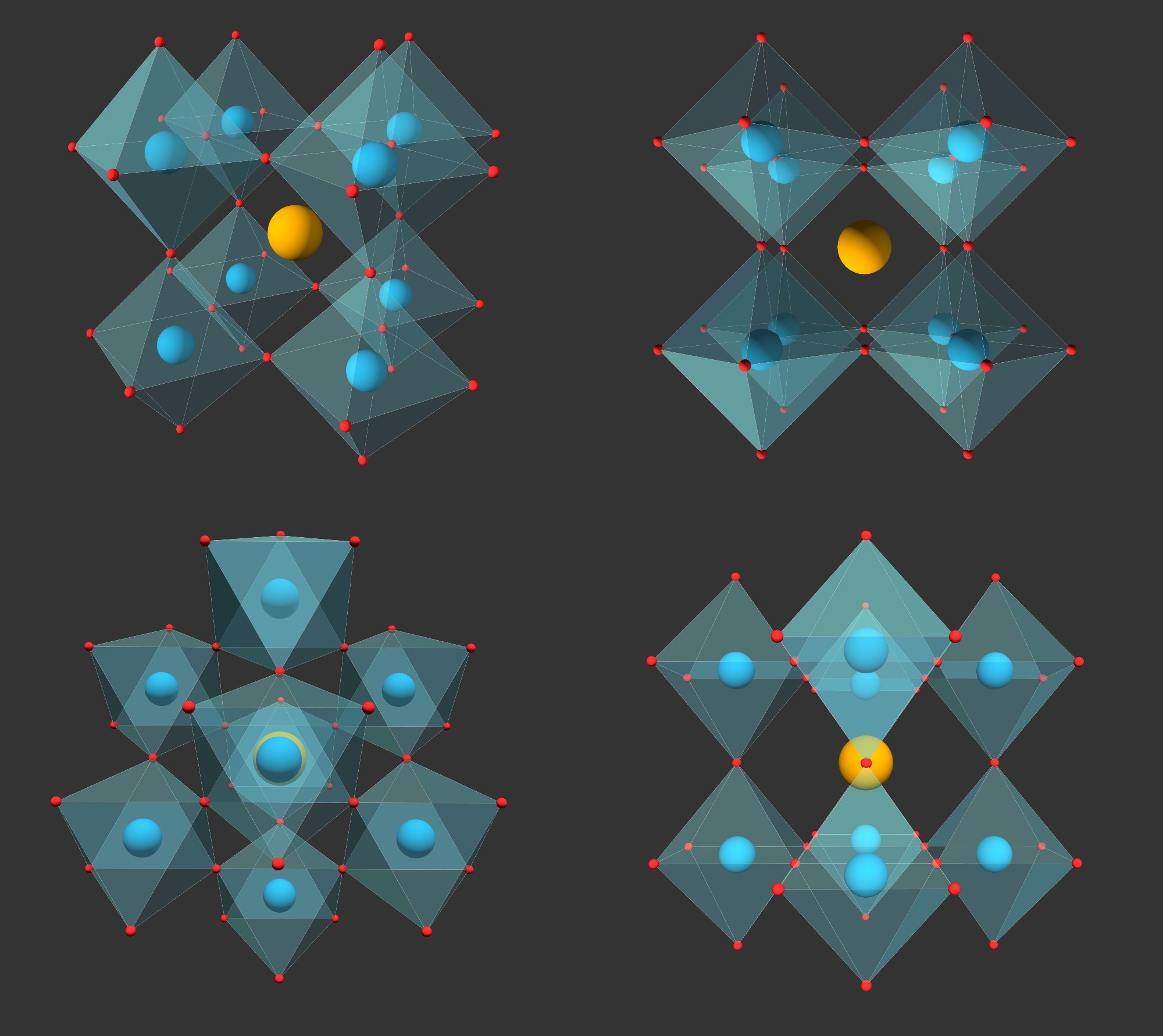
Image Credit: Niethammer Zoltan/Shutterstock.com
Also, the weight is relatively low, and compared to conventional organic semiconductors, the overall performance and the semiconductor properties are much better because we still have this inorganic component in it, but at the same time, it remains flexible. In an early work published in 2018, we demonstrated that they could sustain about 5% to 8% of strain before failure, and that's already beyond the elastic limit of many polymer substrates used for flexible electronics, such as polyimide. This is what endorsed the application of these materials for use in flexible electronics, at least from the mechanical perspective.
You say that these materials are low-cost and scalable. Are there any commercial next steps already in place?
Right now, I think the next steps are overcoming the limiting factors preventing the material from being fully commercialized, which is mainly the stability of the materials. First, there is chemical instability: 3D hybrid organic perovskites have issues in the ambient environment as oxygen, moisture, and UV light can, in combination, attack the structure.
When you go to the 2D materials, the chemical stability is much improved because of the inclusion of hydrophobic organic ligands into the structure. But still, it's not there yet, to fully achieve a 25-year lifetime in service conditions, but at least it's better.
The other instability issue, or bottleneck, is mechanical instability because these materials do tend to fracture. They're subjected to a lot of strain sources during their fabrication and operation, but then the strain can induce a lot of issues, e.g., Ion migration, phase transition, fracture, delamination, etc., and that's our focus. We try to resolve these issues to make sure the new processing technology and new device fabrication have an improved design, such that the material during their service can survive these mechanical loading conditions in order to achieve the desired lifetime in real world for applications.
How do you envision overcoming lingering issues associated with chemical and mechanical instability?
There are already chemists looking into the chemical instability and trying to improve the performance and the thermodynamic stability of these materials. They are coming up with a better chemical design of the ligands to elongate their lifetime in combination with the standard industrial encapsulation technology. On the other hand, new insights are being developed to achieve guidance in materials design to achieve better mechanical robustness. From engineering perspectives, new device architectures can be proposed to mitigate the strain the materials will experience during applications to mitigate their susceptibility to strain-induced failure.
Will you personally be involved with the research to overcome these limitations or bottlenecks?
Yes, I will be involved in resolving the mechanical instabilities of these materials in particular. We will start by investigating the intrinsic mechanical properties of these materials in single-crystal form and checking if the intrinsic properties could meet the application needs. We will further extend the study to polycrystalline forms because the current standard applications and integration of these materials for large-scale device productions rely on polycrystalline films, which will behave differently from the single crystal forms. The study on single-crystal forms will be compared to those from polycrystalline forms to reveal how defects impact the mechanical reliability of these hybrid material films.
We are also looking into how we can produce single-crystalline films in a scalable way without going through the polycrystalline films so that we can integrate the single-crystal films directly with device applications. We want to have a scalable production of single crystalline films without a significant increase in the production cost.
What kind of timescale are you looking at with these improvements?
This is a good question because I believe it probably will come faster from improving the chemical stability of the materials because there is a significant effort underway in that community and the mechanical understanding is slightly lagging behind.
We hope that within the next decade, we can significantly improve 2D hybrids and get them ready for real-world applications. Perovskites are considered to be major candidates for the next generation of photovoltaic materials in order to reach electricity-carbon-neutral goals in the United States by 2035 and then the total economy carbon-neutrality by 2050. So, we hope that by 2035 or even earlier, we can fully resolve these issues associated with 2D hybrid perovskites.
Finally, you mentioned that you had some other research underway. Would you like to tell us a little about some of the other projects that you are working on?
Right now, my focus, as I mentioned earlier, is understanding the surfaces and interfaces for the improved performance of electronics in energy applications. From the perovskite side, we are also looking into the reliability of perovskite materials under the electrical field as well as how they would behave in space-based applications.
Besides that, because we are in the State of Texas, we have a lot of conventional fossil energy production, and these fossil energies generate a lot of byproducts and wastes. We are trying to harvest the fossil fuel production byproducts for green energy applications. For instance, some of these materials we found are organic semiconductor materials, and we can utilize those materials for solar energy applications and other low-power consumption semiconductor applications like memristors. In a nutshell, our slogan is that “we should not waste any waste”.
Where can readers find further information?
Mechanics-coupled stability of metal-halide perovskites
Unveiling the Fatigue Behavior of 2D Hybrid Organic–Inorganic Perovskites: Insights for Long-Term Durability
About Dr. Qing Tu
Dr. Qing Tu is an assistant professor in the Department of Materials Science & Engineering at Texas A&M University (TAMU). He received his B.S. in Theoretical & Applied Mechanics from Peking University in 2011 and Ph.D. in Mechanical Engineering & Materials Science with Outstanding Dissertation Award from Duke University in 2017. During his Ph.D., he also obtained a certificate (minor) in Nanoscience and M.A. in Economics. He then did a postdoc in the Department of Materials Science & Engineering at Northwestern University, during which he was selected as a recipient of American Vacuum Society Electronic Materials & Photonics Division Postdoctoral Travel Award in 2019. He is interested in understanding and engineering the surfaces and interfaces in advanced functional materials (e.g., 2D materials, hybrid organic-inorganic perovskites) from a mechanics perspective to design and optimize their functional properties for applications. He won the ACS Petroleum Research Fund Doctoral New Investigator award and ASME Robert M. and Mary Haythornthwaite Young Investigator Award in 2021.
Disclaimer: The views expressed here are those of the interviewee and do not necessarily represent the views of AZoM.com Limited (T/A) AZoNetwork, the owner and operator of this website. This disclaimer forms part of the Terms and Conditions of use of this website.