Researchers at The Ohio State University have found out a way to control heat using a magnetic field. In their study they have shown that the amount of heat that flowed through a semiconductor could be reduced by 12%, when a magnetic field that was approximately the size of a medical MRI was used.
Researchers at The Ohio State University have discovered how to control heat with a magnetic field. An experiment proved that the phonon—the elementary particle that carries heat and sound—has magnetic properties. Here Joseph Heremans, Ohio Eminent Scholar in Nanotechnology, holds an artist’s rendering of a phonon heating solid material. Artist’s rendering by Renee Ripley. Photo by Kevin Fitzsimons, courtesy of The Ohio State University.
Through their study, the researchers demonstrated that acoustic phonons possess magnetic properties. Acoustic phonons are elemental particles that possess the ability to transmit both sound and heat.
“This adds a new dimension to our understanding of acoustic waves,” said Joseph Heremans, Ohio Eminent Scholar in Nanotechnology and professor of mechanical engineering at Ohio State. “We’ve shown that we can steer heat magnetically. With a strong enough magnetic field, we should be able to steer sound waves, too.”
Heat and sound are not considered to have anything to do with each other, and they are not expected to be controllable by magnets. Heremans agreed to this factor, however, when considered from the quantum mechanical point of view, both heat and sound are expressions of the same form of energy. Hence, if any force is able to control one of them, then it should be able to control the other.
“Essentially, heat is the vibration of atoms. Heat is conducted through materials by vibrations. The hotter a material is, the faster the atoms vibrate.
Sound is the vibration of atoms, too. It’s through vibrations that I talk to you, because my vocal chords compress the air and create vibrations that travel to you, and you pick them up in your ears as sound.
Joseph Heremans, Ohio Eminent Scholar in Nanotechnology and professor of mechanical engineering at Ohio State.
Among the research community, “phonons” and “photons” are considered to be cousins. Both these terms sound quite alike. However, particles of heat and sound are called as phonons, while particles of light are called as photons. These photons have been studied from the time when the photoelectric effect was discovered by Einstein. Phonons, on the other hand have not been studied as much as photons. The present study demonstrates that phonons also possess magnetic properties.
We believe that these general properties are present in any solid.
Hyungyu Jin, Ohio State postdoctoral researcher and lead author of the study
This would mean that heat could be controlled magnetically in conventionally non-magnetic materials if a sufficiently powerful magnet is used. These non-magnetic materials could include plastic, stone, glass and other such materials. In metals, this effect would not be noticeable, as the heat carried by phonons is very, very less compared to the heat transmitted through electrons.
The researchers state that this discovery may not be used practically in the near future, as powerful 7-tesla magnets is presently only being used in laboratories and hospitals. Furthermore, the semiconductors have to be cooled or chilled to a temperature near absolute zero -268°C (-450°F). This cold temperature is required to slow down the atoms, so that the movements of phonons can be detected.
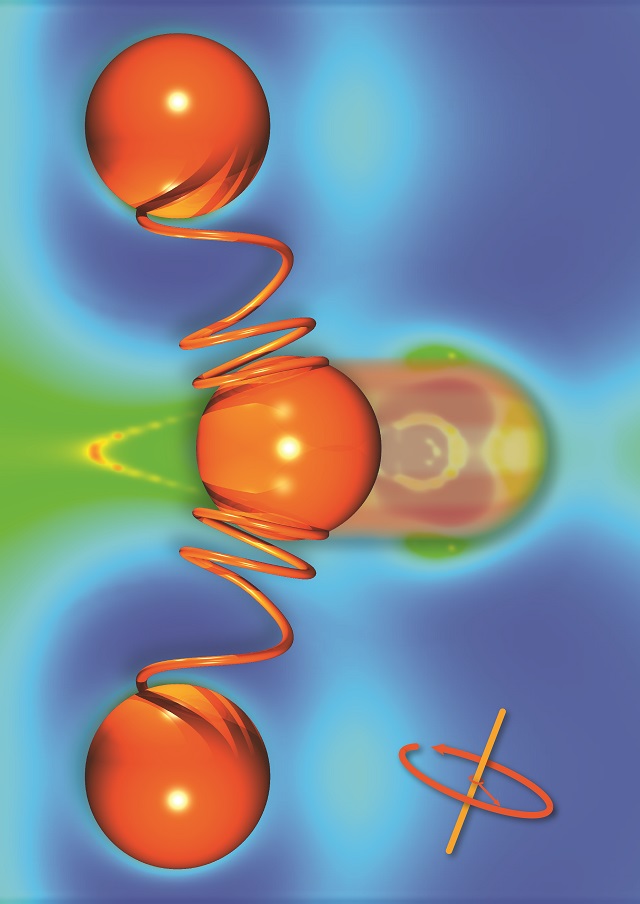
Jin comments that this factor has made it very difficult to perform the experiment. It was also very difficult to take a thermal measurement at this temperature.
Jin shaped a piece of indium antimonide semiconductor into a lopsided tuning fork. The arms of the fork had different widths, with one arm having 4mm width and the other having 1mm width. At the base of the arms heaters were planted. In semiconductors at low temperatures, a quirk exists in its behavior. Due to this factor, the lopsided tuning fork worked. In a normal scenario, the ability of a material to transfer heat would be based on the type of atoms that it is made of. However, at extremely low temperatures, the size of the sample also influences the ability to transfer heat. Hence, heat is transmitted by a larger sample faster than a smaller sample. In this experiment, more heat would be transferred by the tuning fork’s larger arm than by the smaller arm.
Heremans stated the reason for why this happened.
“Imagine that the tuning fork is a track, and the phonons flowing up from the base are runners on the track. The runners who take the narrow side of the fork barely have enough room to squeeze through, and they keep bumping into the walls of the track, which slows them down. The runners who take the wider track can run faster, because they have lots of room.
“All of them end up passing through the material—the question is how fast,” he continued. “The more collisions they undergo, the slower they go.”
The experiment was performed using a 7-tesla magnetic field in turned on condition and also in a turned off condition. The temperature change in the two arms of the tuning fork was measured and the values were subtracted one from the other.
More heat was transferred by the larger arm on the tuning fork than the smaller arm when there was no magnetic field. However, in the presence of a magnetic field, the heat that flowed through the larger arm was reduced by 12%. In this case, the magnetic field made some of the phonons that were going through the material to vibrate in a manner that was out of sync, which led to the phonons to bump into one another. Using computer simulations, Nikolas Antolin, Wolfgang Windl and Oscar Restrepo of the Department of Materials Science and Engineering at Ohio State, identified and quantified this effect.
The researchers found that in the larger arm, the phonons experienced more collisions as the freedom of movement worked against them. Many phonons were knocked off their course. This led to 12% fewer phonons to pass through the material without getting affected.
The team concluded that the particles had to be sensitive to magnetism as the phonons reacted to the effect of the magnetic field. To advance this study, the researchers plan to conduct experiments to find out if they would be able to deflect sound waves sideways using magnetic fields.
The co-authors of this study included Roberto Myers, an associate professor of materials science and engineering, electrical and computer engineer; and Stephen Boona, a postdoctoral researcher in mechanical and aerospace engineering.
The National Science Foundation (NSF), the U.S. Air Force Office of Scientific Research, the U.S. Army Research Office, and the NSF Materials Research Science and Engineering Center at Ohio State have provided funds for this study. The Ohio Supercomputer Center provided the computing resources.
The team of researchers has published their study in the journal Nature Materials.
References