Characterization of surface properties involves taking into account an important parameter known as surface energy. This helps to provide a detailed picture of the energetic situation on the surface, and demonstrates a dependency on various macroscopic properties, such as the flow properties of bulk particles.
Energetic heterogeneity takes place across a wide distribution of surface sites of varying energetic levels. The heterogeneity profile can be represented through an energy distribution function that also provides information on variation in surface properties. A prediction of product properties can be carried out based on a heterogeneity profile, which also constitutes an energy “map” of the material surface.
Flow behavior is a multidimensional property, and flowability is not an inherent material property. However, considering the importance of powder flow, most industries continue to rely on poorly understood and applied flow properties. Different parameters such as hardness, surface texture, shape and particle size can change the rheological properties of powders. Variations in surface chemical environment are often not taken into account while determining the flowability of a particular powder.
In this experimental study, detailed surface energetics of a model pharmaceutical excipient, D-mannitol, were determined using iGC SEA, in order to relate surface energy heterogeneity measurements to powder flow properties owing to surface chemistry changes.
The iGC SEA enables the precise control of the injection size, and hence different amounts of probe vapor can be selected to pass through the sample column to achieve different surface coverages. The surface energy and free energy can be determined upon injecting a of probe vapors at the same surface coverage.
Experimental Method
D-Mannitol Material
D-mannitol (C6H14O6) is a crystalline pharmaceutical excipient used in oral formulations, chewable tablets, moisture sensitive APIs and powder granules. It has a prismatic rod-shaped crystal structure as shown in Figure 1.
.jpg)
Figure 1. SEM image of AR D-mannitol particles
Surface Modification - Methylation
The surface methylation process is carried out using a 5% solution of dichlorodimethylsilane in 1,1,2- trichlorethylene. Under constant reflux, the solution was mixed with AR D-mannitol particles at 80°C for 3 hours. The mixture was continuously agitated to achieve good dispersion.
The methylation reaction, as shown in Figure 2, takes place at the AR D-mannitol surface where the dichloro-dimethylsilane condensates the surface hydroxyl groups. The liquid was decanted off following the reaction, and the samples were dried in a vacuum oven at 80°C for 2 hours.
This treatment ensured the methylation of a large portion of the surface hydroxyl groups following the evaporation of all solvent. Both silanised and AR D-mannitol were then sieved to 75-180 µm particle size fraction.
.jpg)
Figure 2. Chemical reaction scheme for methylation of hydroxyl groups
Powder Flow Measurement
A FT4 Powder Rheometer, was used to conduct the flow tests. Both samples were initially sieved at 500 µm to eliminate any soft agglomerates. Each sample was then placed in a glass jar to be tumbled before testing to achieve a homogeneous state with respect to segregation.
The flow tests, including determination of dynamic, bulk and shear properties, were performed using a 25 mm diameter vessel and a 23.5 mm blade.
Surface Energy Heterogeneity
The analyses were carried out using iGC Surface Energy Analyzer, and both standard and advanced SEA Analysis Software were used for data analysis. Nearly 2 g of samples were packed into individual silanised glass column for all experiments using the SMS Column Packing Accessory.
.jpg)
Figure 3. The iGC-SEA from Surface Measurement Systems Ltd
Samples were run at a series of surface coverages with polar probe molecules and alkanes in order to determine the dispersive surface energy distribution and the specific surface energy distribution. Dorris and Gray methods were used to analyze the dispersive component.
Each column was pre-conditioned at 30°C for 2 hours and 0% RH with helium carrier gas to eliminate any physisorbed water. All the experiments were carried out at 30°C with 10 sccm total flow rate of helium, with the help of methane for dead volume corrections.
Results and Discussions
Dispersive, γsD and specific (acid-base), γsAB surface energy profiles obtained directly from the iGC SEA for both samples are shown in Figures 4 and 5, respectively.
The findings show that AR D-mannitol is energetically heterogeneous, which changes the surface energy as a function of surface coverage. A notable difference between maximum and minimum γsD values, ranging from 37.51 to 52.63 mJ/m2, was also observed.
This finding coincides with previous reports of contact angle measurement and IGC finite concentrations on a native mannitol macroscopic crystal, which has a high level of surface heterogeneity.
A number of -OH groups can found on the AR D-mannitol surfaces. These hydroxyl groups were substituted with – Si(CH3)2 groups after methylation reaction. Through doing this, the silanised surfaces became energetically more homogeneous, with a relatively small mean value of 1.60 mJ/m2, showing an isotropic hydrophobic surface property, as illustrated in the Figure 5.
.jpg)
Figure 4. Dispersive surface energy profiles
.jpg)
Figure 5. Specific (acid-base) surface energy profiles
.jpg)
Figure 6. Wettability profiles
Powder Flow Characteristics
Results prove clear rheological differences between AR D-mannitol and silanised D-mannitol. AR D-mannitol exhibited unstable flow behaviour, showing dramatic variability at low air velocities, as shown in Figure 7.
.jpg)
Figure 7. Flowability energy as a function of aeration
Flowability energy of AR D-mannitol was observed falling from 333.0 to 50.0 mJ. However, silanised D-mannitol has a much lower flowability energy and aerated energy, which implies a less cohesive (more free-flowing) powder property.
The total work of cohesion can be determined using the following equation when irreversible chemical interactions are neglected and only physical interactions are present.
.jpg)
Figure 8 shows a similar trend where AR D-mannitol exhibits variable and higher cohesive strength owing to its more active surface sites and heterogeneous surface property. Variability of AR D-mannitol is also evident in the consolidation test.
This also confirms that silanised D-mannitol is the more free-flowing of the two. Silanised D-mannitol has a slightly lower wall friction angle, which indicates more easy flow over typical metal surfaces, such as those found in storage vessels or in processing equipment.
.jpg)
Figure 8. Thermodynamic work of cohesion as a function of surface coverage
Conclusion
It can be concluded that the methylation process has clearly enhanced the flow properties of D-mannitol under a low-stress environment. Further, change in the surface chemistry, from a heterogeneous surface property to a homogeneous and a low wettability surface property, has reduced the variability in all powder flow properties, achieving more repeatable processing performance.
D-mannitol samples showed excellent powder characteristics with respect to the surface energy distributions determined by iGC SEA. It was also observed that iGC SEA enables effective differentiation of energetic heterogeneity and homogeneity of bulk powders, showing the surface chemical environment.
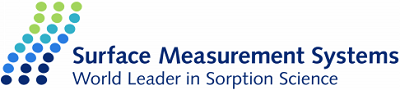
This information has been sourced, reviewed and adapted from materials provided by Surface Measurement Systems Ltd.
For more information on this source, please visit Surface Measurement Systems Ltd.