In practical adsorption processes, the study of the kinetics of adsorption of multicomponent gas mixtures on solid adsorbents plays an important role. Environmental applications include carbon capture and storage (CCS) and geological carbon dioxide (CO2) storage techniques, and also the pre-combustion capture of CO2 from syngas and post-combustion isolation of CO2 from flue gases. The former method includes the storage of CO2 in depleted coal seams and oil and gas reservoirs, whereas the latter approach uses any number of techniques, including adsorption, to remove CO2 from multicomponent gas flows.
There is a wide range of porous materials that can selectively adsorb CO2 over other species. Examples include zeolites, activated carbons, and metal organic frameworks (MOFs), which are being considered for use in CCS technology.
Of primary importance to CCS technology are the kinetics and equilibria of multicomponent adsorption from different mixtures, such as CO2/H2, CO2/CH4 and CO2/N2. This is because the binary combinations effectively represent a range of pre- and post-combustion gas streams; however, the determination of the kinetics of multicomponent adsorption presents a challenging prospect.
In this article, an alternative technique is used to establish the time-dependent displacement of adsorbed CH4 by CO2 in the same activated carbon at ambient temperature. The single component adsorption of CH4 (methane) and CO2 by this material are also measured from room temperature to 45°C, and the respective isosteric enthalpies of adsorption are calculated.
Experimental Details
A Hiden Isochema IMI-FLOW was used to carry out the measurements (Figure 1). The IMI-FLOW is a fully automated manometric-TPD gas sorption analyser, which can be used to determine the materials’ gas sorption properties at pressures of up to 20 MPa. This system analyses the composition of the outlet stream with a built-in quadrupole mass spectrometer. It integrates the capabilities of a high pressure manometric sorption system with the ability to flow gases through the reactor. It also includes two inlet gas streams, and an optional third stream enables binary gas mixture experiments to be carried out, with the third stream used exclusively for the carrier gas.
.jpg)
Figure 1. The IMI-FLOW high pressure manometric-TPD gas sorption analyser
Since helium does not affect the isothermal uptake of other species throughout the flowing experiments, it is typically selected as the carrier. This gas is also used to determine the dead volume of the sample reactor to perform manometric isotherm measurement. The precision of the IMI-FLOW for high pressure manometric isotherm measurement has been significantly improved by ensuring that the system has a minimised internal volume of around 10 cm3. Moreover, the cabinet temperature is controlled to a stability better than ± 0.1 °C, whilst the pressure measurement precision is better than ± 0.05% of the range.
A schematic diagram of the set-up used in this study is shown in Figure 2. It can be observed that the three stream configuration enables the supply of CO2, CH4 and helium to the sample reactor.
.jpg)
Figure 2. A schematic diagram of the IMI-FLOW instrument operating in dynamic flowing mode, with an integrated dynamic sampling mass spectrometer fitted to monitor the downstream gas composition. The inlet flow is regulated by the mass flow controllers (MFCs), while pressure is regulated using the outlet flow controller. The sample sits in a thermally isolated sample reactor with an in-bed thermocouple monitoring the sample temperature.
A mass flow controller (MFC) is used to control each stream, and the gas flow is directed through the packed sample bed. To sample the gas composition at the reactor’s outlet, the instrument is equipped with a Hiden Analytical dynamic sampling mass spectrometer (DSMS). A single MFC regulates the flow rate at the instrument’s outlet beyond the mass spectrometer sampling port, and thus enables accurate control of both the pressure and flow rates through the system.
In this study, the Hiden Isochema IMI-FLOW was initially used in static manometric mode to determine the adsorption isotherms of a single component on a 416 mg BAX950 activated carbon sample using CH4 and CO2. After degassing the sample completely, measurements were carried out at room temperature, 35 °C and 45 °C.
In order to measure the exchange of CH4 and CO2, the measurements were carried out in flowing mode, by keeping a continuous flow of 45 ml min-1 of CH4 into the system during the experiment, and a continuous overall combined inlet flow of 100 ml min-1. Next, the exchange experiment was carried out by decreasing and increasing the inlet flow of CO2 in a stepwise manner, with the remainder of the mixture consisting of helium.
The low inlet flow rate of CO2 was 1.5 ml min-1, whilst the high rate was 45 ml min-1. During the exchange experiment, the DSMS was used to track the gas composition at the reactor’s outlet. The temperature of the sample was also tracked with a thermocouple positioned in the reactor. The measurements were carried out at room temperature (23.5 °C).
Results and Discussion
The low pressure CO2 and CH4 adsorption isotherms determined at 23.5 °C, 35°C and 45°C are shown in Figure 3. Isosteric enthalpies of adsorption calculated from these data using van 't Hoff plots, for uptakes in the range of 50 to 300 micromoles, were in the region from 24.5 to 25.8 kJ mol-1 for CO2 and 19.4 to 20.4 kJ mol-1 for CH4.
These values correspond to the isosteric enthalpies of adsorption at zero coverage of 28.4 ± 0.7 and 23.8 ± 1.1 kJ mol-1, obtained previously by Reid and Thomas for CO2 and CH4 adsorption, respectively, on a carbon molecular sieve. The data set indicates that BAX950 will selectively adsorb CO2 over CH4 because of the higher enthalpy of adsorption for the former.
Moreover, the kinetic data recorded by the Hiden Isochema IMI-FLOW throughout the isotherm measurement demonstrates that the two sorbate species display comparative rates of uptake and release.
.jpg)
Figure 3. Carbon dioxide and methane adsorption isotherms for BAX950 activated carbon measured on the IMI-FLOW at the temperatures indicated in the legend.
.jpg)
Figure 4. The inlet (red trace) and outlet (blue trace) CO2 flows through the BAX950 activated carbon sample at ambient temperature during the cyclic addition of CO2 with a constant CH4 flow of ~45 ml min-1 . The inlet signal is the flow through the CO2 MFC and the outlet signal is the flow detected by the DSMS. A constant net flow of 100 ml min-1 is maintained using helium.
.jpg)
Figure 5. The inlet (blue trace) and outlet (red trace) CH4 flows through BAX950 activated carbon sample at ambient temperature during cyclic addition of CO2 . The inlet signal, at a constant flow of ~45 ml min-1 , is the flow through the CH4 MFC and the outlet signal is the flow detected by the DSMS. The running integral of the displaced CH4 (black trace) is also shown in ml (indicated on the right axis). The in-bed sample temperature (green trace), indicating the exo- and endothermic responses to the CH4 displacement, is displayed on the left hand axis, with units of °C.
Figures 4 and 5 show the data captured in dynamic flowing mode. In Figure 4, it can be seen that there is a delay between the change in the composition of the flow at the inlet stream and the response to this change determined at the outlet stream.
This delay results in a curvature of the outlet flow, indicating that when the inlet flow is increased, the sample adsorbs CO2. This is because the quantified outlet signal would otherwise correspond with the inlet flow. Likewise, when the CO2 flow is decreased, the CO2 signal at the outlet reduces at a slower rate when compared to the inlet flow.
In Figure 5, the upper plot illustrates the CH4 flow during the same experiment. The blue line indicates the constant CH4 flow into the system, whilst the red line shows the flow at the outlet, as determined with the DSMS. The troughs and peaks in the CH4 signal at the outlet evidently show that CO2 has displaced CH4, as the CO2 inlet flow increases and then replaces it when the flow is decreased.
It can be observed that the kinetics of the re-adsorption or replacement of CH4 is slower than the kinetics of the original displacement. This is consistent with the slower CO2 desorption kinetics illustrated in Figure 4, and probably originates from the stronger adsorption affinity displayed by CO2.
During the exchange experiment, the in-bed sample temperature was recorded by means of a temperature sensor positioned on the reactor. The green line in the lower plot of Figure 5 shows the calculated temperature displayed against the left hand axis using units of °C.
This demonstrates that heat evolves as part of the CO2 / CH4 displacement process, and when the CO2 flow is reduced the sample cools down. This corresponds with the isosteric enthalpies of adsorption measured from different isotherms depicted in Figure 3.
Conclusion
The IMI-FLOW manometric-TPD gas sorption analyser was used to determine the kinetics of the displacement of adsorbed CH4 by CO2 on BAX950 by means of a combined mass flow/mass spectrometry technique. Additionally CO2 and CH4 adsorption isotherms in the pressure range from vacuum to 500 mbar and from room temperature to 45 °C were measured.
The data demonstrates that for a given pressure and temperature in the quantified range, the sample adsorbs more amount of CO2 than CH4. This article shows that the IMI-FLOW manometric-TPD gas sorption analyser is capable of determining single component equilibria, as well as the kinetics of multicomponent adsorption by porous materials for viable adsorptive applications.
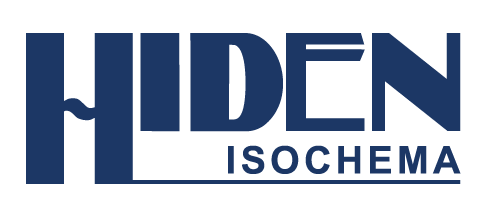
This information has been sourced, reviewed and adapted from materials provided by Hiden Isochema.
For more information on this source, please visit Hiden Isochema.