A fantastic tool for discovering and predicting the activity of catalysts is the determination of the dispersion of active species on catalysts. The quantity of active, accessible particles that are located on a catalyst’s surface can be indicated by the dispersion of active species.
These have direct contact with reactant molecules which have to react and produce a new substance. Consequently, correctly measuring the dispersion of the active species enables you to predict the catalyst’s activity for any given catalytic process.
The technique consists of reducing the active particles in the catalyst at an increased temperature. More often than not, hydrogen is used for this task, either in pure form or as a mixture of H2 balanced inert gas.
Once the reduction is complete, the temperature of the catalyst is increased to room temperature. After this, the catalyst is titrated with the use of a calibrated loop and by dosing known quantities of active gas, usually hydrogen or carbon monoxide.
At the point of saturation, the amount of adsorbed active gas is calculated and related to the accessible active species on the catalyst’s surface. This titration method is easily the most useful method of predicting a catalyst’s activity.
There is a problem, however, when the remaining hydrogen is removed from the catalyst following reduction. This is usually achieved by flowing an inert gas across a sample at an identical reduction temperature. Removing the remaining hydrogen can take a significant amount of time, as much as an hour or sometimes longer, depending on the active particles present and their ability to retain hydrogen.
Once the remaining H2 has been removed, the sample is returned to room temperature while the inert gas continues to flow over the sample. If traces of O2 are present in the inert gas which is being used, the newly produced reduced particles will slightly oxidize, leading to a change in the composition of the accessible particles on the surface that were used to determine the dispersion.
Experimental
For these experiments, a 0.5% Pt/Alumina Micromeritics reference material with a 35% dispersion plus or minus 5 was considered. 100 ml per minute of hydrogen at 400 °C flowed over the sample for an hour in order to achieve the primary reduction.
Once it was reduced, 100 ml per minute of helium was introduced at the reduction temperature for half an hour. After this, the sample temperature was returned to room temperature, at which point pulses of active gas were introduced until saturation was achieved.
In order to follow the masses involved during the analysis, a mass spectrometer Cirrus II was used in the capacity of a detector. Post reduction and following the complete removal of any remaining hydrogen, the first analysis was carried out. The mass spectrometer followed a signal of mass 2 (H2) and pulses of 0.0513 ml were introduced, in order to ensure that the sample was completely saturated.
Illustrated in Figure 1 is the spectrum of H2 where one pulse was adsorbed entirely. Peaks 5 through 9, on the other hand, display complete saturation and were considered when determining the total amount of H2 which was adsorbed (see Table 1).
After this primary analysis was carried out, a flow of 100 ml per minute of helium was used to remove all of the adsorbed H2 at 400 °C. A signal of H2 (mass 2) on the mass spectrometer followed the total removal of H2 at 400 °C.
Immediately following this, the sample was returned to room temperature under the same helium flow. At this point, 0.1 ml of air (containing roughly 0.03 ml of oxygen) was introduced to the carrier gas using a syringe. This was a means of simulating the presence of traces of O2 in case contaminated helium had been used, in order to assess its effect on the dispersion.
In Figure 2, the same pulse technique demonstrates an increased amount of H2 adsorption by the sample. In this instance, the same sample displayed in Figure 1 adsorbed four full injections.
This outcome shows that any traces of O2 which are present in the inert gas, used when cleaning the sample of H2 which remains after reduction, will negatively change the dispersion results. In this instance, the dispersion increased by an approximate factor of 3 (see Table 2).
The procedure just described was repeated using carbon monoxide as the active gas rather than hydrogen. Initially, the passivation procedure outlined above was used to carry out the analysis. The results of CO chemisorption are shown in Figure 3, yielding the correct value of the dispersion which is 35% plus or minus 5 (see Table 3).
Shown in Figure 4 are two spectra that correspond to the CO pulse chemisorption. The one on top illustrates the spectrum of CO which comes from the passivated sample, in the same manner as was used for hydrogen above. After one-third of the primary pulse was adsorbed, the remainder of the peaks indicates saturation.
The spectrum shown in Figure 4 below corresponds to mass 44, indicating the formation of carbon dioxide while CO is pulsed on a passivated or oxidized sample. The final dispersion result suggests that traces of oxygen in the sample are responsible for the overestimation of the dispersion on the Micromeritics reference material (see Table 4).
.jpg)
Figure 1. Pulses of H2 over freshly reduced material.
.jpg)
Figure 2. Pulses of H2 over freshly oxidized material.
Table 1. Quantification and measurement of dispersion on the freshly reduced sample determined by H2 chemisorption.
.jpg)
Table 2. Quantification and measurement of dispersion on the freshly re-oxidized sample determined by H2 chemisorption.
.jpg)
.jpg)
Figure 3. This figure shows the CO pulse chemisorption on the freshly reduced sample.
.jpg)
Figure 4. This figure shows the CO pulse chemisorption on the passivated sample (above), while the spectrum below shows the formation of Carbon Dioxide upon each pulse of CO.
Table 3. Quantification and measurement of dispersion on the freshly reduced sample determined by CO chemisorption.
.jpg)
Table 4. Quantification and measurement of dispersion on the freshly passivated sample determined by CO chemisorptions.
.jpg)
Conclusion
This work indicates that the chemisorption method is extremely sensitive to contamination, particularly for the inert gas which is used in order to remove any excess hydrogen that is still adsorbed by the sample following reduction. The degree to which the result is overestimated is dependent upon the quantity of contaminating substances present in the carrier gas.
Nevertheless, for a good chemisorption analysis, pure gases are necessary. Without these, results have no serious meaning, particularly if they are supposed to correspond to the activity of the catalyst.
In the instance of hydrogen chemisorption, a significant amount of H2 is being adsorbed. While platinum atoms are adsorbing one portion of the hydrogen, a significant quantity of it is being adsorbed or retained by O2 atoms which are situated on the platinum atoms on the solid’s surface.
It can be averred, however, that hydrogen molecules can only be adsorbed without reactions, as demonstrated by the spectra of water which indicates its complete absence. Somehow, however, results from CO chemisorption are different.
Over atoms of platinum, carbon monoxide exhibits higher activity than H2. Carbon monoxide is capable of removing the atoms of oxygen and producing carbon dioxide, as is displayed in Figure 4. This results in a higher dispersion of the material, as shown in Table 4.
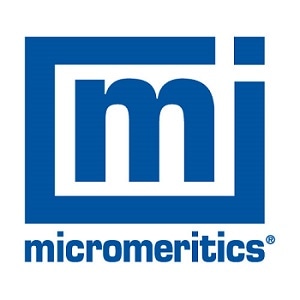
This information has been sourced, reviewed and adapted from materials provided by Micromeritics Instrument Corporation.
For more information on this source, please visit Micromeritics Instrument Corporation.