An outstanding sporty design and superlative performance together with favorable passenger comfort and high efficiency are fundamentals considerations at all times for a car manufacturer like Porsche. Therefore, the new Porsche wind tunnel is a crucially important component of the aerodynamic and aeroacoustic optimization process, which allows evaluation of design and material ideas as well as styling-driven shape decision making. The new Porsche wind tunnel was developed over several years and realized from 2011 to 2014 [14].
Presently, aero-acoustic development can be performed over the full velocity range up to 300 km/h, and due to minimal background noise levels, the localization and precise investigations of sound sources are possible with the use of microphone arrays. The Porsche wind tunnel microphone array system was installed in January 2015 and developed and built in cooperation with GFaI, Berlin.
Basic Array Hardware
The system comprises of four data recorders, three microphone arrays, each equipped with 192 electret microphones and an integrated Full HD camera. In addition, 24 measuring channels are provided to record reference signals and the conditions in the test. A high performance workstation is dedicated to the data acquisition and analysis, run by special controller software, adjusted especially to the Porsche wind tunnel.
To reach a high-degree of precision in the localization of the acoustic sources, a signal sampling frequency up to 192 kHz is realized for each differential transmitted channel and a positional accuracy of ± 1 mm is ensured for all microphones. This accuracy is reached up to maximum speed by a high stability and a low weight of the arrays, which results from a special sandwich construction incorporated into a lightweight frame that provides reinforcement.
Beamforming in a Wind Tunnel
The result created by an array system is known as an acoustic source map; superposed to an optical image or a 3D geometry of the considered item. The calculation of the source map is implemented with the use of beamforming algorithms.
A picture (2D) or model (3D), divided into a grid of points, is used in representing the object that is to be investigated. For 2D applications, the grid is arranged in the scan plane with a known distance to the microphones in the array plane. The evaluation of the different runtimes and amplitude decays of assumed sound sources between every grid point of the scan plane and every microphone of the array plane results in the basic source map (“dirty map”). This fundamental beamforming algorithm can be applied in the time or frequency domain whereat for many years the time domain “delay and sum” algorithm is widely recognized in acoustic signal processing.
In recent years, more highly developed frequency domain algorithms have been made to advance the quality of the maps. In the frequency domain, the vast majority of algorithms are based on the calculation of the cross spectral matrix (CSM). The CSM contains all cross spectra data of the microphone signals and thus all level and phase information, which represents the differing runtimes of the acoustic waves.
With the application of a so-called steering vector to the CSM, including the geometric information and distances - calculating the source map becomes possible. With the formulation of the steering vector, attention can be drawn in the evaluation to calculate precise levels or to a good source localization in the source maps.
The main diagonal of the CSM contains the auto spectra data of the microphone signals, which are regularly overshadowed by the background noise. Therefore, it is possible to better the dynamic range of the source maps by taking away the elements of the main diagonal from the CSM. On the other hand, the energy content and thereby the absolute levels of the source maps are incorrect in that instance.
There are multiple publications in which the varying methods and algorithms with their advantages and disadvantages are in detailed discussion [2], [6], [7], [9], [13].
In a wind tunnel, a shear layer is apparent between the flow region and the area in the plenum without a major flow where the arrays are stationed. The flow surrounding the test object creates sound sources radiating acoustic waves. These waves become strongly affected when passing the shear layer. The shear layer causes diffractions of the acoustic waves, resulting in displacements and distortions in the source maps. For the application of beamforming in wind tunnels to aero-acoustic sources, it is indispensable to consider this shear layer effect, e.g. by a correction according to Amiet [12].
Amiet gives an introduction to a model where the diffraction effects are considered with decent precision by bringing down the shear layer to a flat and infinite thin extent specified by its angle and distance to the array and scan plane.
Missing from consideration in this model are additional scattering effects because of the turbulence in the shear layer, which causes correlation losses of the signals with a deteriorating effect in the evaluation of the phase shifts.
.jpg)
Figure 1: Sketch of wind tunnel shear layer and parameters of the Amiet model.
With the Porsche Array System, the majority of the analyses are performed in the frequency domain with the basis of the CSM with diagonal removal. An Amiet shear layer correction modified by Puhle [8] and a steering vector formulation that focuses on a precise source location is used. The implemented basic algorithms were validated in high detail in the context of an intensive cooperation between GFaI, DLR Göttingen, Volkswagen group research and Porsche.
The Point Spread Function (PSF) may be of use to rate the performance of an array. The PSF is the frequency-dependent array response to an omnidirectional point source with defined location, usually calculated by a simulation. The outcome is also called beam pattern.
In Figure 2, a typical PSF of the Porsche arrays is plotted for the third-octave band of 2500 Hz. The main lobe is representative of the real source, whereas the side lobes are artefacts created by the beamforming algorithms. The dynamic range (DR) is determined by the difference between the level of the main lobe and the highest side lobe.
The beamwidth (BW) determines the width of the main lobe at 3 dB level decrease. Dynamic range and beamwidth are the fundamental indicators for the quality of sound source location and therefore for the capabilities of an array. Both parameters depend strongly on the hardware parameters: the array dimension, the number and layout of microphones and the measurement distance (focus).
.jpg)
Figure 2: Simulated Point Spread Function (PSF), f = 2500 Hz, F = 4 m, DR = 21dB.
Array Dimension
The necessary size of an array is dependent upon the lowest frequency to be considered. The lower the frequency and therefore the larger the wavelength, the bigger the array dimensions to capture enough information of the acoustic wave. Meaning, to measure enough runtime deviations or respectively phase shifts in the frequency domain for the observed wavelength.
To keep the beamwidth to a minimum over the whole frequency range, basically maximum array dimensions with a minimal focus are aspired. Under flow conditions in a wind tunnel, on the other hand, higher frequencies correlation losses grow together with the array dimension and the flow rate. This effect leads to phase errors for microphones located further away.
With all these effects taken into consideration and adapting the arrays to the dimension of the cars to be analyzed, the overall sizes of the Porsche arrays were defined to 5 x 3 m. Additionally, as shown in Figure 3, the central part of the arrays (3 x 2 m) has been developed particularly for the investigation of higher frequencies in a preferential measurement window of 1.5 x 1 m. To gain comparable results, all arrays (left, right and on top) were built with identical dimensions.
.jpg)
Figure 3. Overall array dimension: 5 x 3 m, centre array: 3 x 2 m, extension: 3 x1 m, preferred measurement window: 1.5 x 1 m.
Number and Layout of Microphones
The number of microphones and therefore the number of measurement channels required by the data acquisition system defines a large part of the hardware expenses of an array system restrained by the budget of the project. Otherwise, to attain an excellent dynamic range of the source maps, as much microphones as feasible should be applied. To achieve a balance, each Porsche array was equipped with 192 microphones.
Another significant point, particularly taking in the dynamic range, is the layout of the microphones. A vast amount of energy was used to find the best arrangement of the microphones. Therefore, a brand new optimization strategy was developed and used by Dr. Hartmann from Volkswagen group research.
The optimization undertaken by a generic algorithm combined with a gradient-based search of local minima operates directly to all microphone coordinates – not only to part of the layout parameters. Therefore, for 192 microphones with adjustable x- and z-positions 384 degrees of freedom must be optimized.
The target function for the optimization was created by a rating of weighted sums for each of the criteria below:
- Beamwidth
- Distance of the main lobe to the highest side lobe
- Level of the highest side maximum
- Average of the side lobe levels in the preferred measurement window
Even more restrictions were imposed to focus microphones in the central array. This could be key for the high-frequency region if the use of a shading procedure to the outer microphones could lead to a reduction negative correlation loss effects as previously discussed. In this example, only few microphones would be affected by shading. Future work has been arranged in regard to this topic.
The outcome of the optimization is an irregular microphone layout as can be seen in Figure 4, where simulated source maps are shown in comparison to the optimal symmetric standard layout under consideration.
.jpg)
Figure 4: Simulated source maps, 6 sources with same level, F = 4 m, 192 microphones.
To compare on an identical basis, the number of microphones was extended to 192 for the logarithmic wheel standard layout. The successfully improved dynamic range which was achieved by the optimized layout is clearly seen for all frequencies and particularly apparent in the preferred measurement window.
The only drawback seen when taking into consideration the asymmetric dimension and layout is a marginally asymmetric representation of the sources in the source maps.
In accordance with the array dimension, the number and layout of microphones was found to be the same for all arrays installed in order to receive comparable results.
Measurement Distance or Focus
A framework built for the purpose of integrating the arrays in the wind tunnel was installed on a special rail system to be moveable in flow direction. The fixing to a defined position is achieved by several pneumatic cylinders bracing the whole framework to the walls of the wind tunnel. High system rigidity is realized by this mechanism.
Because of the conditions, a measuring distance of 7 m to the wind tunnel center would have been reached by a direct installation of the arrays to the framework. This corresponds to a focus of approx. 6 m to the scan plan assumed at the side of a car with a typical width. However, as a preferably short measuring distance (focus) is vital for successful outcomes, shorter distances were investigated in a study conducted with 5 microphones mounted at the edges and in the center of the frame with the array dimensions of 5 x 3 m (Figure 5).
.jpg)
Figure 5: Test set-up to determine the optimal measurement distance.
Evaluating the study leads to the result that a minimal measuring distance of 5 m to the wind tunnel center and a focus of approx. 4 m to the assumed scan plane can be achieved. By reducing it further, the microphones close to the collector would be greatly affected by the shear layer. On the other hand, for a focus reduction, an additional mechanical construction is needed. Because of the anticipated improvements in result quality, the decision to implement an array positioning system was taken.
Array Positioning System
The array positioning system for the new Porsche wind tunnel was specially designed. It constitutes of several control arms which connect the arrays rotatable to the framework. It also includes an electric winch system operated by a control unit and a pneumatic locking system to attach the arrays in the parking position reliably (Figure 6 and Figure 7).
Every array can be moved automatically to the measuring or parking position in less than three minutes. A variety of sensors are installed to avert operating errors.
.jpg)
Figure 6: Framework and arrays in measuring position.
.jpg)
Figure 7: Framework in measuring position Arrays in parking position.
All the wiring of the array system is routed through a cable track, to prevent any taping of loose cables and to be ready to perform measurements within a few minutes. As all the system is moveable on the rail system, the preferred measurement window is easy to align to every interesting part of the test object and a parking position can be accessed speedily to fend off any negative effect to aerodynamic testing (Figure 8).
.jpg)
Figure 8: Framework and arrays in parking position.
An almost perfect fixing of the framework to the wind tunnel wall structure, put together with a highly rigid positioning system and the framework, leads to an excellent reproducibility for the array positioning and low displacements and vibrations during the wind tunnel operation, even at maximum speed. For the ideal source localization on a 3D model, this is essential.
Using a FARO Laser Tracker with a measurement accuracy of 0.03 mm at 18 m distance, the deviations of the array positioning looked at a variety of operations and the array displacements within the duration of the wind tunnel operation were measured.
Table 1: Array positioning accuracy.
|
Max. Deviation |
Side Arrays |
0.06 mm |
Top Array |
0.33 mm |
Table 2: Array displacement durin operation.
Flow Speed |
Max. Displacement |
140 km/h |
0.22 mm |
300 km/h |
1.32 mm |
Performance Tests
Setup
To determine the basic array properties, thorough performance tests were undertaken with a 1:1 model of a test vehicle (Figure 9). To provide as much flexibility as possible for analyzing the system, the study was undertaken with generic sound sources, created by loudspeakers (LSP). When in the process of choosing the loudspeakers, much attention was given to meet the following criteria:
- Providing enough power to generate source levels representing real aero-acoustic sources usually analyzed at a speed in the range of 100 and 200 km/h.
- LSP membranes as small as possible for a accurate analysis of the source localization precision.
- No generation of additional sound due to the shape of the LSP themselves.
- The provision of a preferably linear frequency response over a broad frequency range.
Two types of LSP, shown in Figure 10, were discovered that meet the criteria. Type 1, with a membrane diameter of 36 mm, allows the provision of enough power and a nearly linear frequency spectrum between 1 and 10 kHz. LSP of type 2 extends the lower frequency limit to 500 Hz providing almost identical features with a membrane diameter of 50 mm.
.jpg)
Figure 9: Setup of performance test, conducted with 8 loudspeakers.
.jpg)
Figure 10: Loudspeaker used for the performance tests.
Using a suitable signal conditioner and amplifier put together with a newly developed Matlab script, it is possible to run 8 LSP time synchronously with signals independent from each other. The signal levels and frequency characteristics are therefore able to be adjusted by a set of parameters for each LSP separately. The phase relation between the sources can also be changeable.
In all, 16 LSP positions were defined on the side window and the windscreen based on intensive talks between the project partners. For a preferred quality radiation, all LSP are mounted completely flat to the surrounding surface, achieved through the use of a special 1:1 test vehicle model in which the entire glazing is made of foam material instead of glass. However, because of confidentiality reasons, all results reported here are mapped to an image of a current production line vehicle. The LSPs are thereby pictured at identical positions. To keep the focus on the LSP sources the rear view mirrors, one of the most predominant aero-acoustic sources has been omitted from the vehicle model.
For results that can be compared and relied upon, sources emitting the same sound levels and frequency content are vital. Therefore, all LSP were calibrated to radiate the same sound level in one meter distance. Additionally, the frequency spectra were modified to the background noise of the wind tunnel to analyze with a similar signal to background noise ratio for every frequency. All investigations are undertaken based on uncorrelated sources and an adjusted white noise signal.
Source Localization
The source localization precision was looked at first conducting the performance tests. High importance was therefore attached to the comparison of the results without flow and with a flow present, while the shear layer correction was applied.
Figure 11 illustrates the quality of source localisation for the left and the top array with 8 LSP switched on, each emitting sound signals with the identical level and frequency content.
.jpg)
Figure 11: Localisation of 8 uncorrelated sources with the same level at u = 140 km/h,1/3-octave, f = 4000 Hz, DR = 10 dB.
Though, for LSP with the shortest distances, the localization is biased because clear source separation is not possible. Two sources appear as one with a source center in between. The beamwidth and the dynamic range can control this frequency-dependent effect and will be discussed below.
In Figure 12 the localization quality is shown for single LSP sources (sum levels). With the array at the top, the source localization on the windscreen is excellent for the case without a flow and equally as well with a flow of 140 km/h.
On the side window, marginal differences for the localization with the left array can be seen. These difference are most probably caused by small inaccuracies in matching the acoustical and optical calibration of the array as they are almost the same without and with a flow present. In fact, the results also provide confirmation of the perfect functionality of the implemented shear layer correction.
An evaluation which depends on the frequency of the source localization, as depicted in Figure 13 for LSP 1, is indicative of different distances of the located SPL maxima to the LSP membrane center for each 1/3-octave-band. This effect results most likely from a changeable sound radiation by the LSP membrane, with regards to the frequency range of interest. Therefore, LSPs are only suitable for the acoustic source localization to a limited extent.
An optimally performing matching procedure, for the acoustical and optical calibration, which uses a special sound source with a very local sound radiation has already been scheduled. It will improve the quality of the source. It is expected to reach an accuracy of at least +/- 5 mm.
.jpg)
Figure 12: Localization of a single source without and with a flow present, DR = 1 dB.
.jpg)
Figure 13: Frequency dependent source localization of LSP 1 without flow (Scale 1:1, printed on DIN A4)
Beamwidth
The beamwidth is the basis for the source separation in a beamforming map. The smaller the beamwidth, the sharper a source appears in the map and the better a separation is possible. Based on the basic array parameters, dimension and focus, according to Sijtsma [11], at a level decrease of 3 dB an estimation of the beamwidth can be calculated.
In Figure 14, a typical beamwidth is plotted for a frequency of 800 Hz to show the manner of the beamwidth determination in the beamforming map at a 3 dB level decrease.
Due to the irregular design of the Porsche arrays, the horizontal beamwidth is different from the vertical one and thereby casusing sources to appear asymmetric in the maps.
In comparing the estimated and measured beamwidth, depicted in Figure 15, a very good agreement and a strong frequency dependency of the beamwidth is unveiled. Figure 16 depicts the beamwidth of the Porsche arrays measured at a variety of flow speeds.
.jpg)
Figure 14: Determination of the beamwidth, 1/3-octave, f = 800 Hz, DR = 3 dB.
.jpg)
Figure 15: Estimation and measurement of the horizontal and vertical beamwidth.
.jpg)
Figure 16: Measured beamwidth of the Porsche arrays.
Thus far, no ready reasoning could be discovered for the marginally improved beamwidth at 200 km/h in the mid frequency region. The seen higher values for the beamwidth at higher frequencies and flow speeds are most likely caused by the correlation loss effects for distant microphones with the use of a large array. An already planned study will investigate these effects in detail.
Source Separation
For an aero-acoustic analysis of a car, an individual and detailed evaluation of every independent source is vital. Therefore, a good separation of the sources is needed, basically dependent upon a preferably small beamwidth.
Figure 17 shows the way of determining the source separation, by a 3 dB criterion or a visual evaluation. The slight level differing between the two sources, emitting equal level are caused by the used steering vector formulation, chosen to give the optimal localization accuracy.
.jpg)
Figure 17: Determination of the source separation for LSP 5 and LSP 6 without flow, 1/3-octave, DR = 3 dB.
Figure 18 demonstrates the capability of the Porsche arrays to separate sources with identical levels for analysis without a flow. With the use of simulations, the limitations of separation were found by reducing step by step the source distance for each 1/3-octave-band, evaluating the 3 dB criterion and the visual impression. These results are compared with the measurements, representing the realized discrete distances between the loudspeakers.
.jpg)
Figure 18: Separation of horizontal arranged sources on the side window without flow, 1/3-octave.
Without a flow, the correlation between simulations and measurements is excellent and the visual source separation is thereby almost the same as the horizontal beamwidth. With a flow present, the source separation at higher speeds and frequencies is also marginally disturbed by the afore-mentioned correlation loss effects.
Using advanced post-processing techniques, for example algorithms like CLEAN SC [12], can make notable improvements to the source separation. Weaker sources may become less easy to detect by dominant sources and their side lobes. By a sequential subtraction of the dominant sources and their correlated fractions from the dirty map, the originally masked sources can be unveiled.
Dynamic Range
The dynamic range (DR) of an array is predominantly defined by how many microphones and how they are arranged. For the Porsche arrays, a notably high DR could be achieved using 192 microphones, irregularly arranged in the best possible layout as previously described.
Figure 19 and Figure 20 demonstrate the measured DR with regards to the sound radiation of LSP 6 for a large scan plane of approx. the size of the arrays (5 x 3 m) and for the optimized window of 1.5 x 1m. The results are then compared to the DR of a simulated monopole sound source (Point Spread Function) located at the position of LSP 6. All analyses are undertaken in the frequency domain based on the CSM with diagonal removal which includes a positive effect to the DR.
The differences that can be seen are most likely caused by the inhomogeneous local sound generation of the loudspeaker membrane and in addition by reflection and damping effects which perform real measurements. Further developed algorithms such as CLEAN SC [12] can be of use to increase the DR.
.jpg)
Figure 19: Beamforming map for LSP 6 without flow, 1/3-octave, f = 6300 Hz, DR = 25 dB.
.jpg)
Figure 20: Dynamic range of the Porsche arrays without flow, 1/3-octave.
Typical Results
In Figure 21 and Figure 22 the valuation of a production line vehicle is depicted for the left side array and the top array. All anticipated sound sources and the improvements applying the CLEAN SC algorithm are easily seen for every frequency.
Small differences of the source location in this overview plots are caused by a focus adjusted to only one scan plane defined in a particular distance to the array. Sources that are not located in this plane are calculated with a wrong focus which leads to a marginally biased source representation in the beamforming map. Therefore, to stop this effect from occurring, the source mapping on a 3D model of the test object will be set up as a base method. Thus, the correct focus is under consideration for every single grid point, and for every source with an assumed location on the test object surface.
Summary and Outlook
A unique microphone array system was developed for the new Porsche wind tunnel and installed. An extremely excellent basic acoustic performance and fast easy-to-use data acquisition was achieved by using a complex design, perfectly attached to the wind tunnel walls, assembled with very high system rigidity.
On the basis of a study with LSP sources, the capability of the arrays was shown, and the limitations were able to be discovered.
To significantly improve the data analysis, further advanced evaluation techniques, like 3D-, differential- and correlation-beamforming [3], [10], have been implemented and an investigation of a frequency dependent shading procedure for distant microphones was begun.
.jpg)
Figure 21: Evaluation of a production line vehicle by the left side array.
.jpg)
Figure 22: Evaluation of a production line vehicle by the top array.
References
[1] Amiet, R. K.: “Refraction of Sound by a Shear Layer”, Journal of Sound and Vibration, Vol. 58, No. 2, pp. 467-482, 1978.
[2] Ehrenfried, K., Koop, L.: “A comparison of iterative deconvolution algorithms for the mapping of acoustic sources”, AIAA paper 2006-2711, 2006.
[3] Döbler, D., Puhle, Ch., Heilmann, G.: “Correlation of high channel count beamforming measurement of a car in a wind tunnel using CLEAN-SC”, inter.noise conference, San Francisco, 2015
[4] Dougherty, R. P. “Extension of DAMAS and Benefits and Limitations of Deconvolution in Beamforming”, AIAA Paper 2005-2961, 2005.
[5] Lechner, W.: “Evaluierung der Leistungsfähigkeit einer akustischen Kamera hinsichtlich Dynamik, Auflösung und Lokalisierngsgenauigkeit im neuen Porsche Windkanal”, Bachelor Thesis, TU Berlin, 2015.
[6] Oerlemans, S., Broersma, L., Sijtsma, P.: “Quantification of airframe noise using microphone arrays in open and closed wind tunnels”, NLR Report NLR-TP-2007-799, 2007.
[7] Oerlemans, S.: “Detection of aero-acoustic sound sources on aircrafts and wind turbines”, PhD thesis, University of Twente, Enschede, 2009.
[8] Puhle, C.: “Über die Scherschichtkorrektur nach Amiet”; Technical Document GFaI, 2014.
[9] Sarradj, E.: “Three-Dimensional Acoustic Source Mapping with Different Beamforming Steering Vector Formulations”, Advances in Acoustics and Vibration, Volume 2012.
[10] Schmidt, S., Döbler, D.: “Visualization of small design modifi ations using differential beamforming”, inter.noise conference, San Francisco, 2015.
[11] Sijtsma; P.: “Experimental techniques for identification and characterisation of noise sources“, Advances in Aeroacoustic and Applications, VKI Lecture Seri s, 5:15-19, 2004.
[12] Sijtsma, P.: “CLEAN based on spatial source coherence”, AIAA Paper 2007-3436, 2007.
[13] Sijtsma, P.: “Acoustic beamforming for the routing of aircraft oise”; NLR-Report, NLR-TP-2012-13, 2012.
[14] Stumpf, H., Röser, P., Wiegand, T., Pfäfflin, B., Ocker, J., Müller, R., Eckert, W., Kroß, H.-U., Wallmann, S.: “The new aerodynamic and aero-acoustic wind tunnel of the Porsche AG”, 15. Internationales Stuttgarter Symposium, 2012.
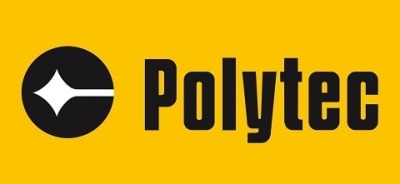
This information has been sourced, reviewed and adapted from materials provided by Polytec.
For more information on this source, please visit Polytec.