High-temperature nanoindentation is a popular method with numerous applications in materials science, bioscience, coatings and additive manufacturing. The method is generally utilized for the investigation of the mechanical properties of materials at small length scales.
Since mechanical properties are usually temperature-dependent, the development of high-temperature nanoindentation will have a significant impact relative to industrial applications where materials are used or processed at high temperatures.
The Development of Nanoindentation
Indentation tests cover a range of techniques for identifying the mechanical properties of materials.
These tend to follow the same format: indent a test material with a probe until an impression is created, and characterize the mechanical properties of this material dependent on the size of the load, displacement of the indenter and sample, as well as the impression’s geometry.
While macro-scale indentation methods (such as the Vickers or Rockwell hardness tests) can offer a practical measure of the relative hardness of various materials, precision measurements can only be accomplished using small loads and indentations.1 Nanoindentation takes this technique to its logical conclusion.
Nanoindentation is identified by loads that range from a few micronewtons to a few millinewtons distributed across an area that ranges from just a few square nanometers to a few square micrometers.
Nanoindentation produces deformations on the material’s surface that are so minuscule that they cannot usually be resolved using optical microscopy.
Nanoindentation provides a number of advantages over macro-indentation. Indentations on this scale facilitate the ability to measure the mechanical properties of films, coatings and small samples to be without influence from the underlying substrate.2
Additionally, nanoindentation can be utilized for the investigation of microscale features or variations in material properties, such as the various constituent phases of complex microstructures.3
The early nanoindentation systems were first developed in the 1970s, driven by an increased interest in microelectronics and biomaterials, which involved the study of small-volume samples and thin films.4
The nanoindentation is now in widespread use for the characterization of materials, mostly due to the fact that many systems now have the capacity to automate analysis and therefore only require a clean, flat (and relatively small) sample to work with.5
As techniques were developed in the early 1990s (including the Oliver and Pharr analysis), measurements can be obtained directly from a force-extension curve without the necessity to characterize the impression itself.6
A traditional nanoindentation procedure can produce statistically evaluated measurements of an extensive range of mechanical properties automatically, including hardness and elastic modulus.
High-Temperature Nanoindentation
As with the majority of mechanical properties, the hardness and elastic modulus measurements acquired using nanoindentation are based on temperature-dependent features.
In order to accurately portray the mechanical behavior of engineering materials with high operating or processing temperatures, high-temperature nanoindentation must be performed.
High-temperature nanoindentation entails controlled heating of the sample and indenter to extreme temperatures – temperatures around 800°C are generally used. High-temperature nanoindentation is often conducted in a vacuum to avoid sample oxidation and other atmospheric effects.
High-temperature nanoindentation exhibits numerous technical challenges, such as sample oxidation, temperature drift and heat management.
However, the advancement of high-temperature nanoindentation systems suchlike the Alemnis High-Temperature Module (HTM), has facilitated a prevailing use of high-temperature nanoindentation.
Thus, the popularity of high-temperature nanoindentation continues to grow, and it is now a conventional method of probing temperature effects on material properties.
Applications of High-Temperature Nanoindentation
High-temperature nanoindentation offers a new approach for fundamental materials research. The exceedingly localized nature of high-temperature nanoindentation implies that it can be utilized for comprehensive investigations of the kinetic features of a material’s behavior.
High-temperature nanoindentation has been used effectively and with success to characterize a wide range of temperature-induced material effects.
These include phase transitions in semiconductors like Si and Ge7, the crystallization performance of bulk metallic glasses8, stress-strain of P91 steel9 and the origins of atomic-level plasticity in solids.10,11
High-temperature nanoindentation is also well-suited for use in combination with several imaging techniques (including SEM and TEM) or measurements of electrical measurements to general a common understanding of the behavior of materials at the nanoscale.12
In the latest developments, these composite in-situ techniques facilitate real-time investigation of temperature effects on microstructure and the interactions between mechanical, thermal and electrical effects at the nanoscale.
Impact testing and multicycle indentation are also being utilized to study the resistance a material demonstrates towards deformation at various temperatures.
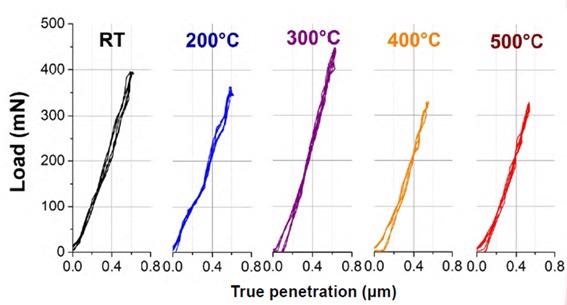
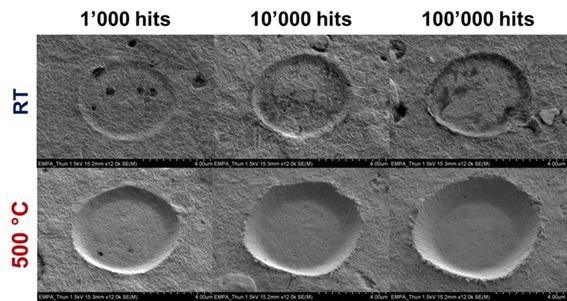
Load-displacement curves obtained between room temperature and 500°C for impact tests on a CrN coating with 10k impacts. Shown is an overlay of curves from the 20th to the 40th impact and residual imprints from room temperature and 500°C nano-impact tests (1k and 100k) using a diamond flat punch. Image Credit: Alemnis AG
High-temperature nanoindentation systems are well adapted to rapid thermal cycling because nanoindentation testing utilizes small-sample volumes. The investigation of thermo-mechanical fatigue of thin films that are of significant interest in the electronics and semiconductor industries exploits such characteristics.5
As well as facilitating compressive testing, high-temperature nanoindentation systems developed by Alemnis also enable tensile testing. This allows for the high-temperature dynamic testing of microscale samples and features, such as those yielded by focused ion beam or lithography.
Alemnis High-Temperature Nanoindentation Systems
The Alemnis Standard Assembly (ASA) is the most dynamic indentation system in the world, offering a modular platform for developing nanomechanical testing methods that are application-specific.
Modifying the ASA with one of four high temperature modules produces a strong, high-performance and economical system for high temperature nanoindentation applications.
Alemnis high-temperature modules facilitate heating up to 200°C in air and up to 1000°C in vacuum. The system contains three thermal sensors with < 1°C resolution and two independent temperature control loops for precision temperature setting and regulation.
The result is a high-temperature nanoindentation environment that can be controlled with great accuracy while maintaining a short temperature stabilization time.
Contact Alemnis today to find out more about ASA’s capacity for high temperature nanoindentation applications.
References
- Fischer-Cripps, A. C. Introduction to Contact Mechanics. (Springer Science & Business Media, 2000).
- Fischer-Cripps, A. C. A review of analysis methods for sub-micron indentation testing. Vacuum 58, 569–585 (2000).
- Fischer-Cripps, A. C. The IBIS handbook of nanoindentation. (Fischer-Cripps Laboratories, 2009).
- Poon, B., Rittel, D. & Ravichandran, G. An analysis of nanoindentation in linearly elastic solids. International Journal of Solids and Structures 45, 6018–6033 (2008).
- Wheeler, J. M., Armstrong, D. E. J., Heinz, W. & Schwaiger, R. High temperature nanoindentation: The state of the art and future challenges. Current Opinion in Solid State and Materials Science 19, 354–366 (2015).
- Oliver, W. C. & Pharr, G. M. An improved technique for determining hardness and elastic modulus using load and displacement sensing indentation experiments. Journal of Materials Research 7, 1564–1583 (1992).
- Chavoshi, S. Z. & Xu, S. Temperature-dependent nanoindentation response of materials. 14.
- Nieh, T., Iwamoto, C., Ikuhara, Y., Lee, K. & Chung, Y. Comparative studies of crystallization of a bulk Zr–Al–Ti–Cu–Ni amorphous alloy. Intermetallics 12, 1183–1189 (2004).
- A. Ruiz Moreno, P. Hähner, F. Fumagallia, V. Haiblikova, M. Conte, N. Randall, Stress−strain curves and derived mechanical parameters of P91 steel from spherical nanoindentation at a range of temperatures, Materials & Design, 194, (2020) 108950
- Quantitative insight into dislocation nucleation from high-temperature nanoindentation experiments | Nature Materials. https://www.nature.com/articles/nmat1429.
- Choi, I.-C., Brandl, C. & Schwaiger, R. Thermally activated dislocation plasticity in body-centered cubic chromium studied by high-temperature nanoindentation. Acta Materialia 140, 107–115 (2017).
- Nili, H., Kalantar-zadeh, K., Bhaskaran, M. & Sriram, S. In situ nanoindentation: Probing nanoscale multifunctionality. Progress in Materials Science 58, 1–29 (2013).
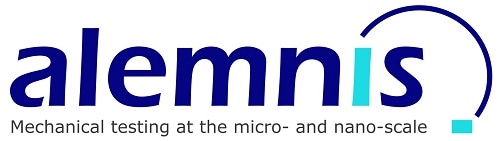
This information has been sourced, reviewed and adapted from materials provided by Alemnis AG.
For more information on this source, please visit Alemnis AG.