Oxford Instruments is at the forefront of understanding and solving the questions posed by the quantum computing revolution, for example, creating cryogenic environments or high-precision manufacturing of devices that operate within those environments.
For almost four decades, Oxford Instruments Plasma Technology has worked with leading researchers to find process solutions for a diverse array of device structures and materials.
Utilizing a process library of over 7,000 recipes that spans material compounds and elements, Oxford Instruments Plasma Technology works in close collaboration with the quantum community, offering solutions designed to minimize losses stemming from device processing.
This article reviews the effect of surfaces and interfaces on losses. It also demonstrates ways in which plasma processing can be utilized to rein in such losses.
Leading researchers and companies in the field of quantum computing, including the likes of Will Oliver,1 John Martinis,3 David Pappas,2 and Rigetti Computing,4 have researched the effects of surface engineering on superconducting resonator and qubit performance.
Quantum computing literature in this area indicates that the performance of the superconducting LC resonator (one of the most basic elements of quantum computers) can be considerably impacted by the slightest details of device fabrication.
Surfaces are known to be vital in the manufacture of a wide range of high-performance quantum appliances. These include ion traps, which can suffer from surface electric field noise,4 color centers,5 quantum dots,7 and photonics.
While this article focuses on superconducting quantum devices, strategies and principles employed to enhance interfaces for these systems may also be relevant to a wide range of quantum and classical devices.
Click Here to Read the Full Whitepaper
References
- Calusine, Greg, et al. "Analysis and mitigation of interface losses in trenched superconducting coplanar waveguide resonators." Applied Physics Letters 112.6 (2018): 062601.
- McRae, Corey Rae Harrington, et al. "Materials loss measurements using superconducting microwave resonators." Review of Scientific Instruments 91.9 (2020): 091101.
- Wenner, J., et al. "Surface loss simulations of superconducting coplanar waveguide resonators." Applied Physics Letters 99.11 (2011): 113513.
- Nersisyan, Ani, et al. "Manufacturing low dissipation superconducting quantum processors." 2019 IEEE International Electron Devices Meeting (IEDM). IEEE, 2019.
- Kaviani, Moloud, et al. "Proper surface termination for luminescent near-surface NV centers in diamond." Nano letters 14.8 (2014): 4772-4777.
- Brown, Kenneth R., et al. "Materials Challenges for Trapped-Ion Quantum Computers." arXiv preprint arXiv:2009.00568 (2020).
- Eriksson, M. A., S. N. Coppersmith, and M. G. Lagally. "Semiconductor quantum dot qubits." MRS bulletin 38.10 (2013): 794-801.
- Wang, Chen, et al. "Surface participation and dielectric loss in superconducting qubits." Applied Physics Letters 107.16 (2015): 162601.
- O'Brien, William, et al. "Superconducting caps for quantum integrated circuits." arXiv preprint arXiv:1708.02219 (2017).
- Houck, Andrew. “New material platform for superconducting transmon qubits with coherence times exceeding 0.3 milliseconds” [Conference Presentation]. APS March Meeting 2021.
- Lei, Chan U., et al. “Multilayer Microwave Integrated Quantum Circuits” [Conference Presentation]. APS March Meeting 2021.
- Melville, Alexander, et al. “Dielectric Loss in Titanium Nitride and Aluminum Superconducting Resonators” [Conference Presentation]. APS March Meeting 2021.
- Bruno, A., et al. "Reducing intrinsic loss in superconducting resonators by surface treatment and deep etching of silicon substrates." Applied Physics Letters 106.18 (2015): 182601.
- Ahles, Christopher, et al. “A Dry NF3/NH3 Plasma Clean for Removing Si Native Oxide and Leaving a Smooth Si Surface”. 2018 MRS Spring Meeting & Exhibit, April 2-6, 2018, Phoenix Arizona.
- Premkumar, Anjali, et al. "Microscopic Relaxation Channels in Materials for Superconducting Qubits." arXiv preprint arXiv:2004.02908 (2020).
- Banerjee, Archan, et al. “Process Optimization for Superconducting Resonators via Identification and Mitigation of Interface Loss Mechanisms” [Conference Presentation]. APS March Meeting 2021.
- Place, Alex PM, et al. "New material platform for superconducting transmon qubits with coherence times exceeding 0.3 milliseconds." arXiv preprint arXiv:2003.00024 (2020).
- Zhang, Xiaohang, et al. “A study of intrinsic dielectric loss of tantalum based superconducting quantum circuits” [Conference Presentation]. APS March Meeting 2021.
- Zichi, Julien. NbTiN for improved superconducting detectors. Diss. KTH Royal Institute of Technology, 2019.
- Melville, Alexander, et al. "Comparison of dielectric loss in titanium nitride and aluminum superconducting resonators." Applied Physics Letters 117.12 (2020): 124004.
- Morozov, Dmitry, et al. "Design and characterisation of titanium nitride subarrays of kinetic inductance detectors for passive terahertz imaging." Journal of low temperature physics 193.3 (2018): 196-202.
- Burkard, Guido, et al. "Superconductor–semiconductor hybrid-circuit quantum electrodynamics." Nature Reviews Physics 2.3 (2020): 129-140.
- Knoops, Harm CM, et al. "Status and prospects of plasma-assisted atomic layer deposition." Journal of Vacuum Science & Technology A: Vacuum, Surfaces, and Films 37.3 (2019): 030902.
- Sandberg, Martin, et al. "Etch induced microwave losses in titanium nitride superconducting resonators." Applied Physics Letters 100.26 (2012): 262605.
- Tolpygo, Sergey K., et al. "Advanced fabrication processes for superconductor electronics: Current status and new developments." IEEE Transactions on Applied Superconductivity 29.5 (2019): 1-13.
Acknowledgements
Produced from materials originally authored by Russ from Oxford Instruments.
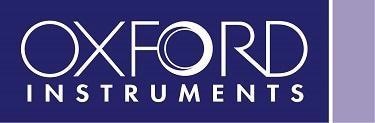
This information has been sourced, reviewed and adapted from materials provided by Oxford Instruments Plasma Technology.
For more information on this source, please visit Oxford Instruments Plasma Technology.