In this Interview, AZo Materials is talking to Tim Lumb and Dr Kevin Nott to discover more about the benchtop NMR techniques and the applications and benefits that they can bring to the laboratory.
Could you start by providing a brief overview of Time Domain NMR and benchtop NMR spectroscopy and what sort of things these techniques can measure?
Traditional high field NMR uses superconducting magnets, cryogens such as liquid helium and liquid nitrogen, and an array of specialist facilities, including an appropriate air supply. These systems also require trained experts to maintain and supervise their use.
Benchtop NMR instrumentation does not suffer from these disadvantages because they employ permanent magnets and are therefore cryogen-free. These instruments are typically compact, requiring just a mains power supply, allowing them to be located in standard laboratory environments.
Two types of benchtop NMR instrumentation are available. High-resolution NMR spectroscopy uses high magnetic field strength and homogeneity to visualise chemical information or spectra from a wide variety of nuclei, including hydrogen, carbon, sodium and phosphorus - all of which are of interest in food science. These samples must be either liquid or in solution.
Low-resolution Time Domain NMR (TD-NMR) uses a lower magnetic field strength and homogeneity to accommodate large sample sizes therefore, it is not possible to acquire chemical information. However, there are very few restrictions as to the size and type of sample that can be analysed because the radio frequency radiation penetrates the whole of sample which may be solid, liquid or typically a mixture of both.
Time Domain NMR analysers are typically used by food companies in process and quality control, as well as in testing laboratories. The main requirement for both time-domain and spectroscopy is that the sample does not affect the magnetic field or prevent the radiofrequency radiation from penetrating it.
How does TD-NMR differ from spectroscopy, and what are some of the advantages of this method in the food industry?
NMR spectroscopy requires the Fourier transformation of the NMR free induction decay signal to produce an NMR spectrum. In contrast, TD-NMR requires separation and measurement of the signals from the different decay components.
These are already well resolved in some samples, and we can read the signals directly from the composite signal. This is typical of many time-domain NMR applications, but we may need to use fitting software to deconvolve the composite signal into different components in other samples. This is usually referred to as ‘relaxometry.’
The free induction decay signal consists of various components, which can be distinguished by their phase or state. The signal from solid and crystalline components decay fast, while the signal from solid-like components, such as residual and bound moisture (concentration below 10%) have a slower decay.
In comparison, oils and fats have the slowest decay, meaning that these can be measured when signals from all the other components have decayed.
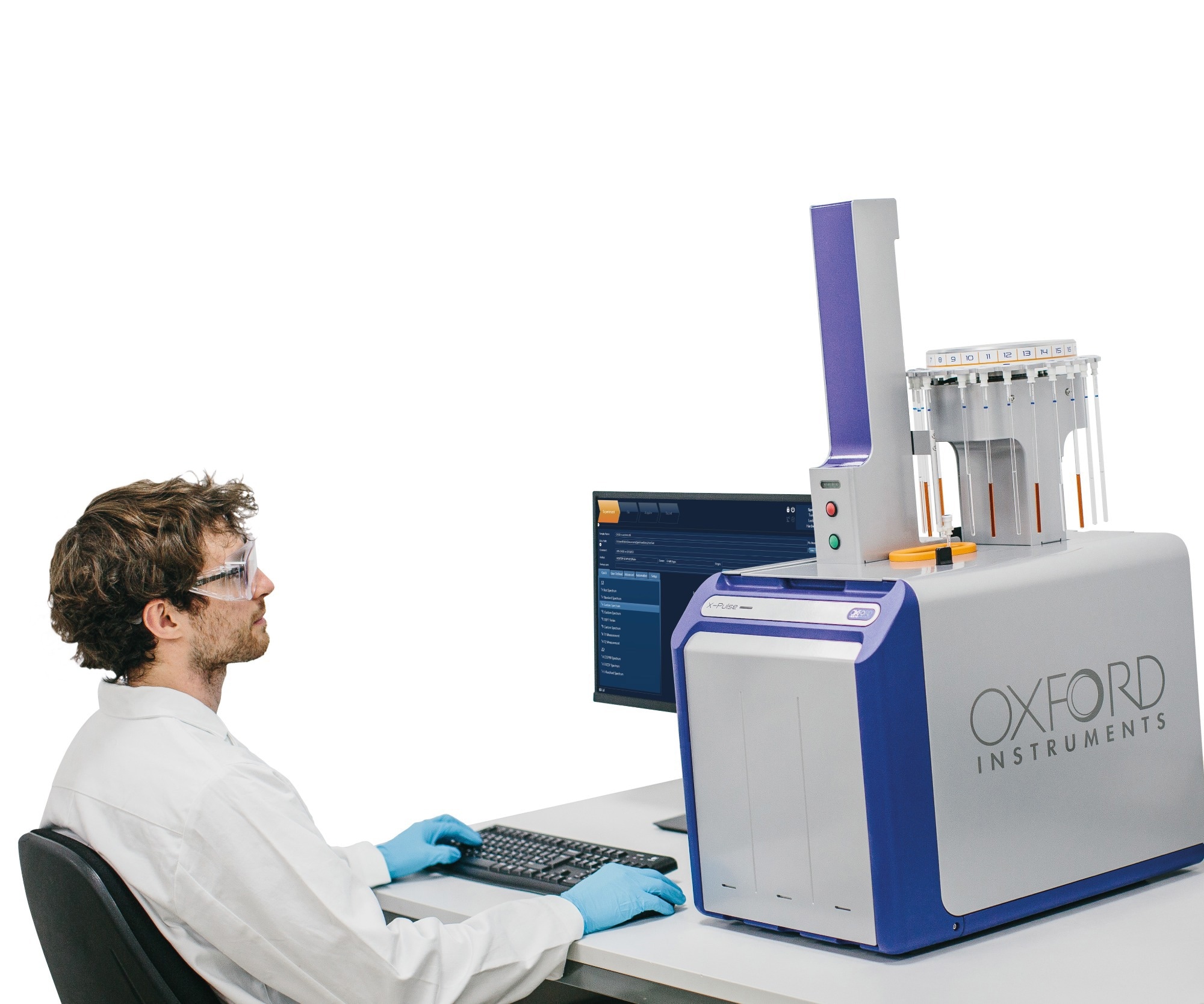
Image Credit: Oxford Instruments Magnetic Resonance
TD-NMR has been used as a solvent-free method to measure oil and fat in food for decades.
It is primarily used for quality control but is also used to help set up and monitor production lines for snack foods, including nuts, as well as chocolate and cocoa derivatives used in their manufacture.
It can also analyse milk powder, infant and nutritional formula, food ingredients and animal food, all without the need for drying. Foods with high moisture content, such as meat, fish, dairy and many processed foods, must be dried beforehand.
For example, TD-NMR can be used according to the Nordic Committee on Food Analysis (NMKL) method for the measurement of dried fish products, whereby the instrument is simply calibrated using a cod liver oil sample.
There are other instances where it is possible to calibrate using pure oil, particularly when working with processed foods and foods which have a high-fat content. For example, snack foods can be calibrated using a sample of the pure oil used in the frying process.
One of the major advantages that TD-NMR has over other secondary techniques is that it is largely insensitive to colour, particle size and composition. NMR calibrations are also linear, so results are often valid even if the sample for analysis lies outside of the existing concentration range.
TD-NMR can distinguish solids from liquids, meaning it is also possible to measure solid fat content at different temperatures, which relates to the melting properties of the edible oil or fat.
Besides pure oils, fats or blends, solid fat content is normally measured for both dairy and non-dairy spreads such as butter and margarine, as well as fats used in the bakery and confectionery industries.
The melting profile of fat relates to its sensory and physical properties, which is important for its eventual end-use. For example, shortenings tend to have higher solid fat contents across the temperature range to ensure the baked goods in which they are added are solid but not greasy at ambient temperatures.
In contrast, margarine has lower solid fat content to ensure that it is soft and spreadable when it is removed from the fridge.
TD-NMR is internationally recognised for the measurement of solid fat, and there are AOCS, ISO and IUPAC official methods for the direct method.
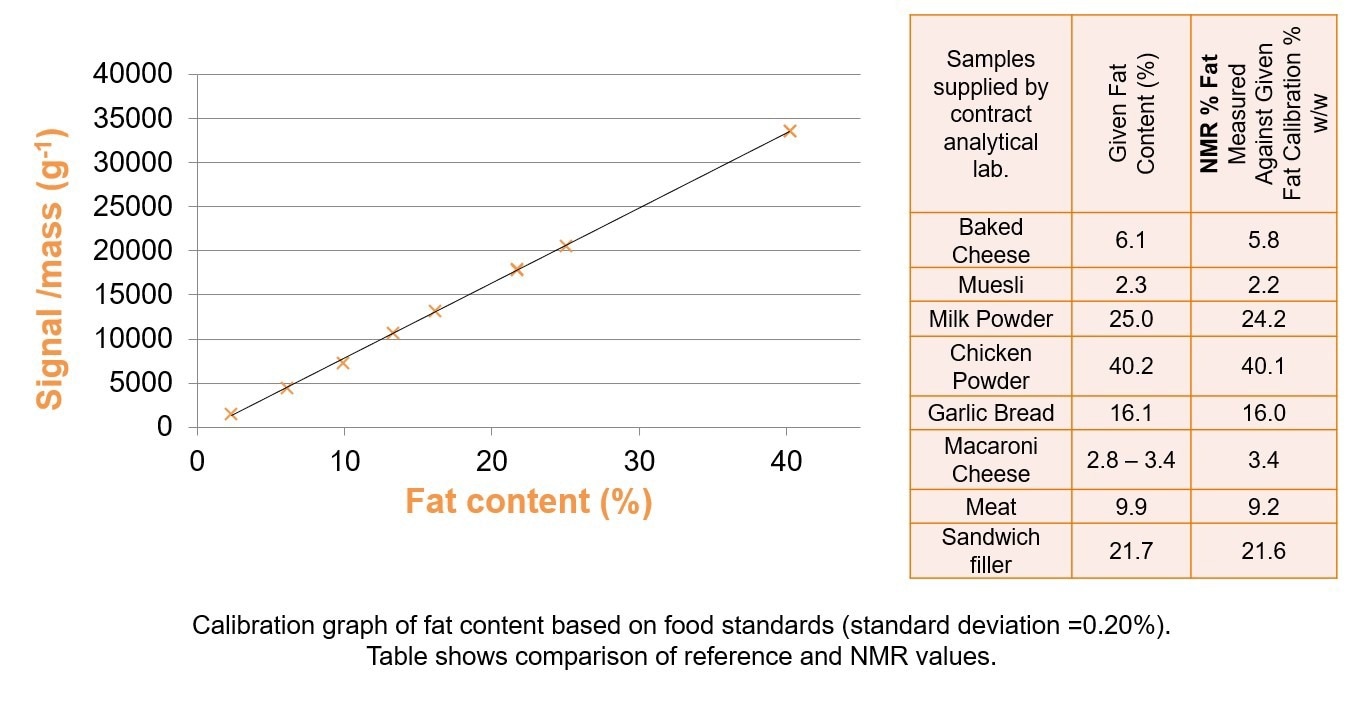
Image Credit: Oxford Instruments Magnetic Resonance
What are the capabilities of benchtop NMR spectroscopy systems, and what are their advantages?
Benchtop NMR spectroscopy systems are often compared to their high field counterparts. These are much less expensive at just a fraction of the capital cost to purchase, and they are extremely cheap to run since there are no cryogens used; the latter is particularly advantageous due to recent helium shortages. Furthermore, only minimal expertise is required to maintain and run the instrument.
Despite only being available for the past ten years, benchtop NMR spectroscopy is now a relatively mature field. The powerful combination of improved magnet design, modern electronics and computing power has led to the development of a number of industrial applications, particularly in the food sector.
Oxford Instruments has been collaborating with Dr Kate Kemsley and her group at the Quadram Institute in the UK to investigate whether benchtop NMR spectroscopy of hydrogen nuclei could provide compositional information suitable for labelling.
Edible oils are largely made up of triglycerides. These are comprised of a glycerol backbone bound to three fatty acids which differ in length and number of unsaturated bonds between and within oils.
With regard to food composition, the main interest is determining the proportions of monounsaturated, polyunsaturated and saturated fatty acids. Spectra will tend to exhibit clear differences and similarities between oil types, most notably in terms of the band height or areas.
The glyceride region is similar across all oils and can function as an internal standard and chemical shift reference. Olefinic bands arise from any hydrogen atom attached to carbon involved in a double bond.
Bis-allylic bands are formed by hydrogen atoms attached to carbons located in between pairs of double bonds. Kemsley was able to directly calculate the number of monounsaturated, polyunsaturated and saturated fatty acids in oils by unpicking these other signals.
This allowed the team to find a good correlation between the results from the NMR method and those from gas chromatography for a set of test oils. They also found that the correlations between predicted and actual values are high for monounsaturated and polyunsaturated fatty acids.
The correlation for saturated fatty acids was slightly less but still good. This covered a narrower concentration range compared to the others.
The outcome of this work was the development of a five-minute test on the purity of oils. This analysis method has since been embedded in a simple software tool, which returns the monounsaturated, polyunsaturated and saturated fatty acid values for each spectrum.
The team was also able to distinguish and quantify the concentration of omega-3 fatty acids.
As well as compositional data, benchtop NMR can also provide a fingerprint that can potentially be used for authentication. Kate Kemsley's group investigated whether benchtop NMR spectroscopy could provide a solution for fraud detection in the coffee sector.
Two different species of coffee make up nearly all commercial production. Arabica is more in demand, but it is harder to grow, while Robusta produces higher yields but has much poorer sensory properties meaning it is used in lower grade coffee products. This is also reflected in the trading price difference.
The limit of detection for Robusta in Arabica coffee is between 1% and 2%, which is at the border between what could be accidental contamination and that which is fraudulent.
Kemsley's group found that they could identify Robusta coffee by the presence of a 16-O-methylcafestol peak at 3.16 ppm chemical shift. It was previously thought that this cafestol derivative was present in Arabica, but the team found this was not the case by analysing a variety of beans known to be authentic. They also produced a software tool to carry out this type of analysis.
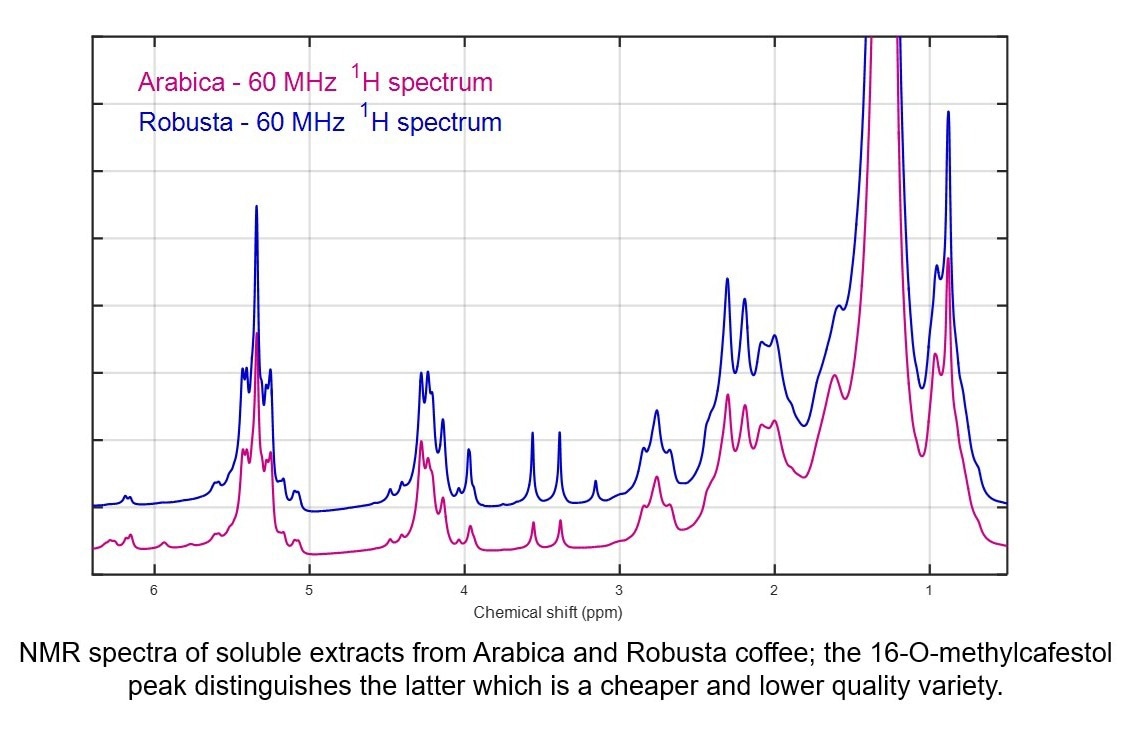
Image Credit: Oxford Instruments Magnetic Resonance
What are some of the factors that have led to TD-NMR's growing popularity and acceptance?
We are now seeing TD-NMR accepted as the standard method across most of Europe. It is also gaining popularity in Asia, Australia and Latin America. We are seeing the acceptance of NMR for total fat analysis in the USA, and a lot of standard methods now make reference to TD-NMR methods for products such as meat and dairy.
ALS Global began working with Oxford Instruments on TD-NMR in 2008. Prior to that, we used a traditional acid hydrolysis method for the analysis of total fat. That method is a long process with a number of steps.
First, we had to take a chunk of sample and weigh this into a vessel. We then added concentrated hydrochloric acid and boiled this for several hours before washing and drying this to remove all remaining aqueous acid. We then boiled petroleum ether and allowed this to condensate and drip back through the sample, extracting the fat with it.
Once this process had finished, we would dry away the solvents by boiling these into the atmosphere or collecting the solvent for reuse in further extractions. Finally, we would weigh the remaining oil.
The way that TD-NMR works in this process highlights exactly why laboratories love this technique.
Does TD-NMR have any notable limitations? How can these be overcome?
Water can pose a challenge to TD-NMR, particularly if containing greater than 10% water which must be dried away.
There are two popular techniques for doing this. The first involves drying samples in an oven for around 16 hours. This method can be used to dry many samples at once, and while rapid drying methods are available, these tend to only be suitable for one sample at a time.
As well as knowing how much fat is in a product, many manufacturers also want to know how much water is present. Weighing the samples again after drying allows manufacturers to calculate the moisture content of samples.
Depending on how the NMR is set up, analysis typically involves taking between eight and 16 specific measurements over 20 seconds and reporting an average result.
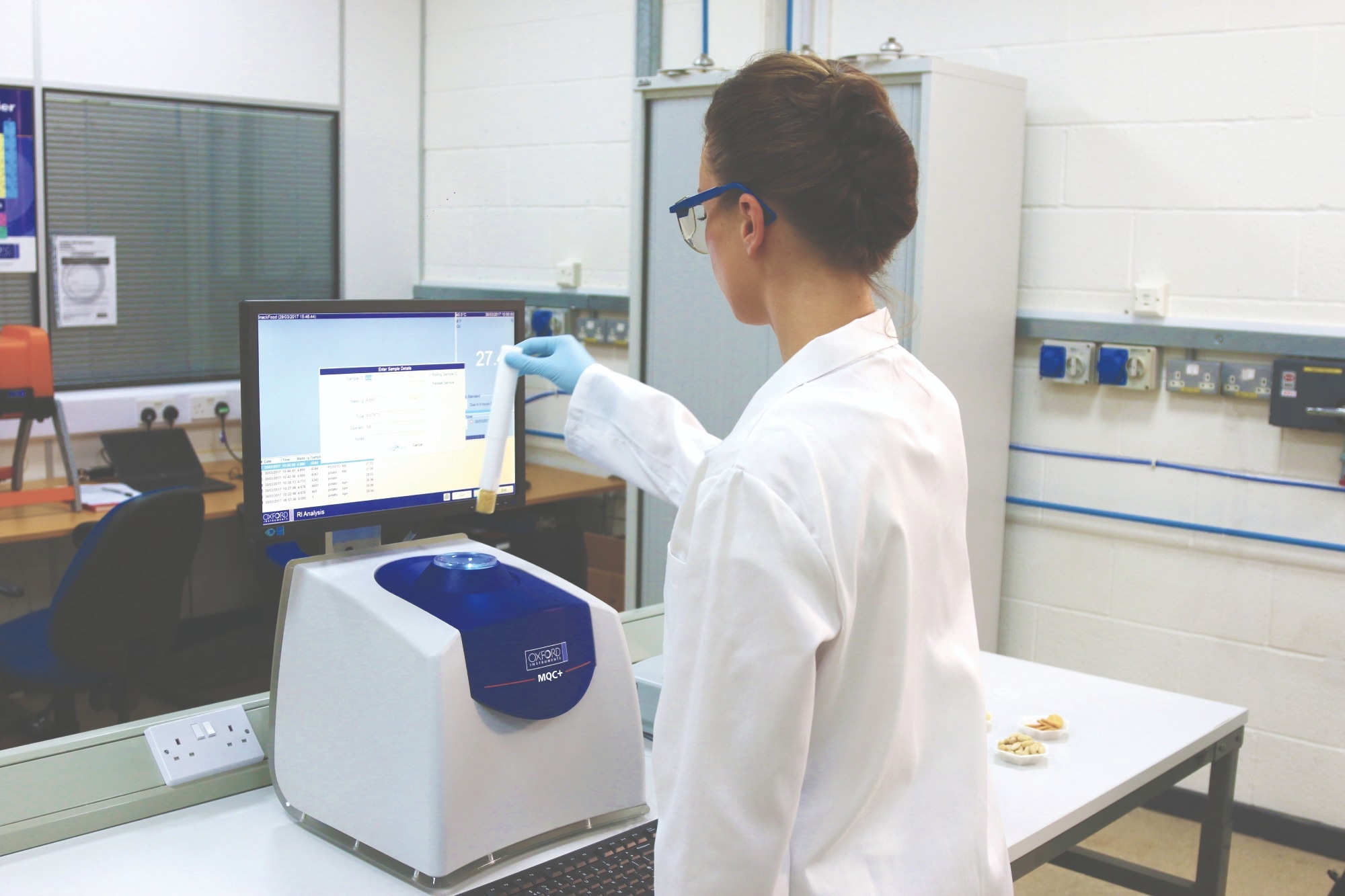
Image Credit: Oxford Instruments Magnetic Resonance
What impact has TD-NMR had on the wider industry?
TD-NMR's impact on the food industry has been centred around cost control. Since the introduction of these methods, we have seen labour and consumable costs increasing massively. The cost to the laboratories only goes one way.
However, if we look specifically at total fat analysis, which is a key parameter for all food manufacturers and retailers, we can see that the cost of that analysis has decreased significantly over that same period of time.
Prices for total fat analysis are around 25% of the price it was in 2008 due to this change of method. Using acid hydrolysis for the majority of samples would not allow the industry to control costs effectively, increasing costs throughout the supply chain to the final consumer.
The precision of TD-NMR has also had a major impact on the industry. This precision is important because the trueness or bias of the method will vary considerably based on how the calibration is set up. Regardless of how that calibration is set up, the precision of NMR will always be better than a manual method.
We have seen improved quality in proficiency tests and a reduction in RSD (Relative Standard Deviation) over time as more laboratories have moved across to NMR.
TD-NMR has greatly benefitted laboratories over the past ten years, but there is so much more we can do with this technology, particularly as the industry starts using benchtop NMR spectrometers operating at 60 MHz.
For example, TD-NMR can tell us how much total oil and fat is in a material, but we can use benchtop NMR spectroscopy to characterise the oil and look at the fat-soluble compounds and other components within it. These can include individual fatty acids or groups of fatty acids within foodstuffs, as well as sterols, stanols and cholesterols.
We can already see and characterise these components, but as the technology advances, we will be able to quantify those using advanced hydrogen and carbon-13 NMR techniques.
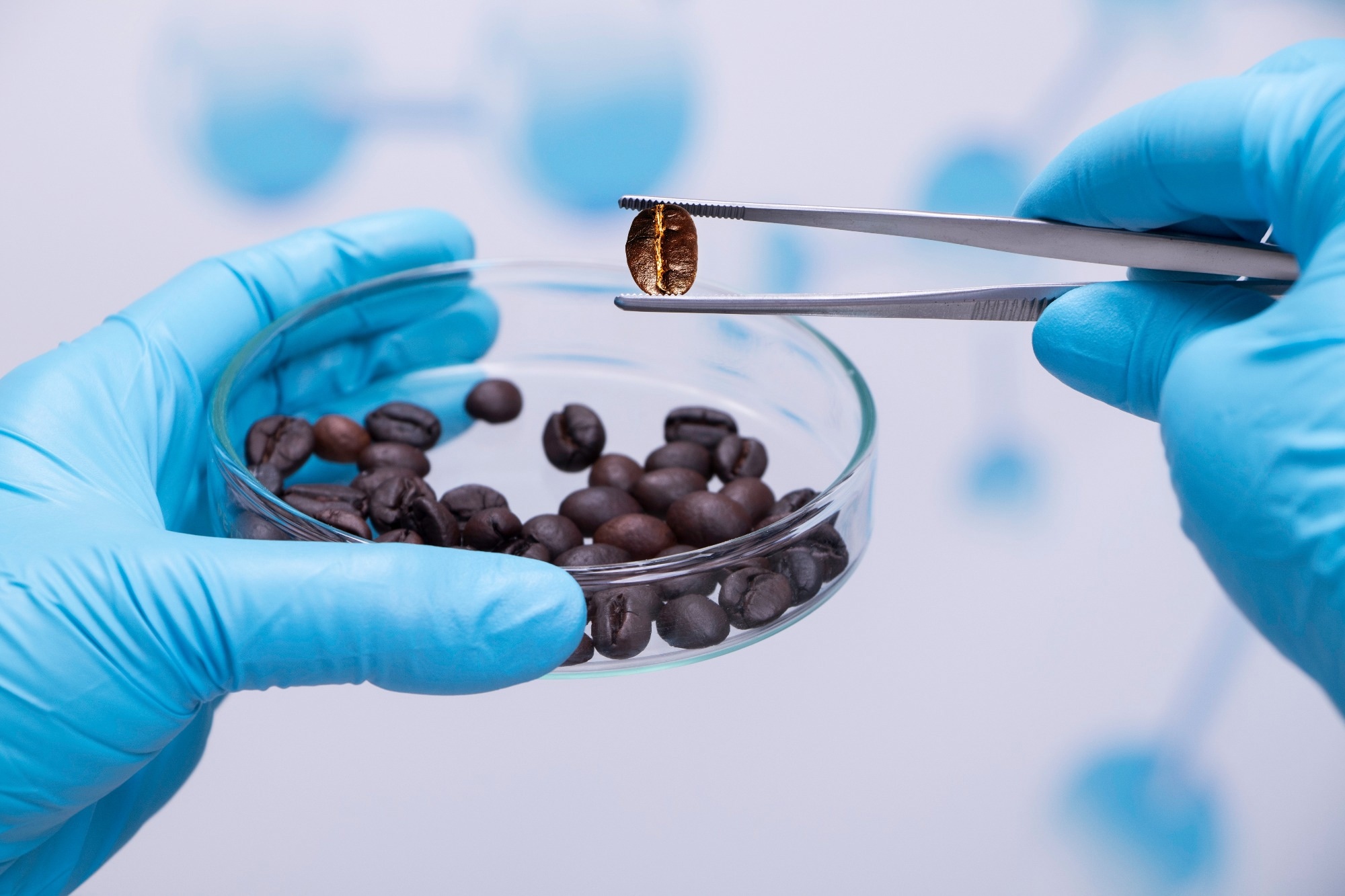
Image Credit: Tonhom1009/ Shutterstock
Could you elaborate on the use of benchtop NMR spectroscopy in detecting food fraud?
The real scope and potential of benchtop NMR remain in its capacity for characterisation, and that is where it is a vital tool in authenticating food products and looking at fraud.
One example study used benchtop NMR to authenticate argan oil. The study began by examining the spectra for pure oil and looking at how oil with a small amount of adulteration caused the spectra's peaks to change.
These peaks will be different depending on the nature and degree of contamination, allowing us to determine the likelihood of contamination of a certain substance.
This is useful when performing a root cause analysis into an adulteration issue to determine if this stemmed from deliberate adulteration or accidental contamination within the factory itself.
Beyond oils, benchtop NMR also offers a useful tool for verifying the authenticity of coffee, as we have discussed, and of high-value herbs and spices. Many herbs and spices contain essential fatty compounds, so there is the potential to use benchtop NMR to verify the authenticity of these.
For example, herbs like Oregano include aromatics and phenolics that are very specific to that particular spice. If this was cut with olive leaves, we would expect to either see a reduction in some of those aromatics or something starting to appear from another source. This approach can also be used with other spices such as cumin and paprika.
One of the most significant applications of NMR and high-field NMR is the detection of honey adulteration. Honey and urine are related in terms of their potential for solvent suppression on NMR.
A lot of work has been done in clinical settings looking at identifying specific compounds in urine in order to diagnose diseases by looking at particular metabolites that should or should not be present in urine.
One of the most challenging historical issues with benchtop NMR has been the problem with the size of the water peak. In some matrices, it is hard to see anything else when the water peak is extremely large.
However, modern solvent suppression on NMR allows us to see all of these analytes of interest, including organic acids, proteins and sugars.
These components are also found in many foodstuffs, and some of the most common uses for high-field NMR are looking at these particular components.
When we look at a typical honey spectrum on a high field spectrometer, we can see acids, proteins and sugars. If we can access this level of detail using benchtop NMR, this could open up means of verifying foodstuffs’ authenticity using a number of other parameters beyond those fatty components.
About Tim Lumb and Dr Kevin Nott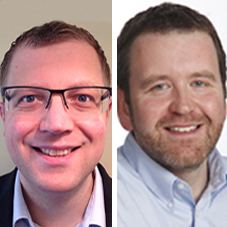
Tim Lumb - ALS Global : Food Chemistry Technical Manager (UK), Food Chemistry Technical Co-ordinator (Europe) and Life Sciences Innovation Co-ordinator (Europe). Tim has been working in the food industry for 15 years, starting as a Laboratory Technician studying nutrients in a range of foodstuffs. After holding various management and technical roles both in the UK and Europe, Tim now holds the position of Food Chemistry Technical Manager (UK), Food Chemistry Technical Co-ordinator (Europe) and Life Sciences Innovation Co-ordinator (Europe) at ALS. In these roles Tim is responsible for all technical development for the food business in the UK – advising producers, manufacturers and retailers of the most appropriate means to verify that the food they produce and sell is safe, nutritious and authentic. Tim has been working with NMR instruments from Oxford Instruments since 2008.
Dr Kevin Nott - Oxford Instruments: Product Manager. Kevin has worked at Oxford Instruments since May 2005. Kevin was previously at the University of Cambridge where he researched into non-medical applications of TD-NMR and MRI.
This information has been sourced, reviewed and adapted from materials provided by Oxford Instruments Magnetic Resonance.
For more information on this source, please visit Oxford Instruments Magnetic Resonance.
Disclaimer: The views expressed here are those of the interviewee and do not necessarily represent the views of AZoM.com Limited (T/A) AZoNetwork, the owner and operator of this website. This disclaimer forms part of the Terms and Conditions of use of this website.