Copper alloys are commonly used across manufacturing and have extensive application in electronics and engineering. Elemental composition is the factor that governs the properties of the many copper alloys available and accordingly the applications they are suitable for. Knowing the composition of a copper alloy and how it relates to the properties is essential.
Metrological institutes such as BAM (German Federal Institute for Materials Research and Testing) provide reference materials that are useful in proving accuracy of the elemental composition for applied analysis methods.
This application note will show how Micro-X-ray fluorescence spectroscopy (Micro-XRF) can be used to enhance the analysis of copper alloys by using XTrace, the micro-focus X-ray source from Bruker, coupled to a scanning electron microscope (SEM) and working in conjunction with its energy dispersive spectrometer (EDS) functionality.
Sample
The Micro-XRF results achieved for the CuZn39Pb3 alloy (ERM®-EB375, BAM) certified mass fractions are shown in Table 1. The certificate provides further 10 elements in the 100 ppm (mg/kg) mass fraction range.
Table 1 Measured and certified values in mass% normalized with standard deviations for the elemental composition of ERM®-EB375. The 30 spectra were acquired on different sample areas using the ESPRIT 2 object mode with image extension.
|
Cu |
Zn |
Pb |
Fe |
Ni |
Sn |
Mn |
Co |
Measured
mean value |
59.306
± 0.528 |
37.790
± 0.267 |
2.394
± 0.599 |
0.202
± 0.018 |
0.111
± 0.010 |
0.185
± 0.073 |
0.007
± 0.004 |
0.006
± 0.004 |
Certified
value |
58.32
± 0.050 |
38.02
± 0.080 |
2.90
± 0.030 |
0.207
± 0.004 |
0.1053
± 0.0015 |
0.20932
± 0.00240 |
0.0222
± 0.0002 |
0.01964
± 0.00028 |
Measurement Conditions
A SEM was equipped with a Bruker QUANTAX microanalysis system including an XTrace source, which is a focused X-ray photon source with a Rh anode, in combination with a 30 mm² active area silicon drift detector XFlash® 6I30 with an energy resolution of 123 eV for Mn Kα.
The SEM was utilized for electron excitation experiments, whereas the XTrace was used for photon excitation procedures. The XFlash® SDD is able to detect X-rays generated by sources, of both electron and photon excitation.
EDS spectra were acquired within the parameters of 25 kV and 300 s live time. Micro-XRF spectra were attained at 50 kV, 600 µA and 10 s real time and 300 s live time.
SEM/EDS Characterization
Fig. 1 shows a BSE image of the polished specimen. The sample is heterogeneous and therefore does not fulfil the demands for a EPMA (electron probe micro analysis) reference material defined by the International Standards Organization standard ISO 14595.
.jpg)
Figure 1. SEM image (BSE) of the polished ERM® -EB375 specimen. The pink squares and the crosshair display the areas analyzed with EDS.
Fig. 2 shows the EDS spectra of the emphasized areas in Fig. 1. The heterogeneity of the sample gives discrepancies between the acquired spectra and quantification. Aside from the main elements detected, namely Cu, Zn and Pb, other elements were detected as well (Ni, Fe, and Sn) at low concentrations.
.jpg)
Figure 2. Logarithmically plotted EDS spectra of the 5 analyzed positions shown in Fig. 1. The difference in the intensities is visible.
Micro-XRF Analysis
Due to the heterogeneous nature of the specimen, XRF is actually more suitable to define the alloy ‘s mean composition than EDS. This is because XRF is able to analyze a larger proportion of the sample. Fig. 3 shows a spectrum attained with 300 s live time at various specimen tilt angles to adjust the refracting lattice planes. The crystal-like nature of the sample is responsible for diffraction reflexes in the spectrum that overlay the characteristic X-ray lines. The diffraction reflexes mask the Mn, Fe, Co and Ni-K lines and makes it very difficult to determine their intensity.
.jpg)
Figure 3. Micro-XRF spectrum of ERM® -EB375 measured at tilt angles of 0°, 10°, and 20°. Unlabeled peaks are diffraction peaks.
The XTrace provides a filter wheel designed with three primary filters prepared from specific metal foils. By placing these filters between the X-ray source and the sample, diffraction peaks can be effectively suppressed to provide a cleaner spectrum. A 15 µm thin Titanium filter was used in one of the alloy analyses to suppress diffraction reflexes above the Ti-K edge at 4.964 keV. The spectrum resulting from this measurement is shown in Fig. 4. The deconvolution of peaks in this spectrum is shown in Fig. 5. It highlights how effective this method is in detecting elements with no interference of diffraction peaks.
.jpg)
Figure 4. Micro-XRF sum spectrum calculated out of 30 spectra measured with a 15µm Ti filter.
.jpg)
Figure 5. Deconvolution result of the Micro-XRF spectrum in Figure 4. Unlabeled peaks are diffraction peaks.
Considering the samples heterogeneity, 30 Micro-XRF point spectra were attained on several sample areas with the ESPRIT 2 object mode using image extension to cover a larger area. The acquisition period for each spectrum was 10 seconds and a standardless fundamental parameter method was used for quantification.
Table 1 displays the mean value and standard deviation of the quantification results for the point spectra compared to the certified values. The quantification results shown are largely in agreement for the majority of elements. Co and Mn could actually be identified only by using Micro-XRF, as its concentration was below the limit of detection (LOD) of the EDS method. Even for Micro-XRF, the element Pb showed heterogeneity. The quantification result of Pb varied between 1.328 and 3.756 mass% and meant the Cu and Zn content was also variable. Table 2 shows the quantification results of four spectra out of 30 attained on different sample positions. These four example spectra display a broad variation in the concentrations of Pb, Cu and Zn.
Table 2 Measured values in mass% normalized of four exemplary Micro-XRF analyses for the elemental composition of ERM®-EB375.
|
Cu |
Zn |
Pb |
Fe |
Ni |
Sn |
Mn |
Co |
Analysis 3 |
58.758 |
37.812 |
2.938 |
0.210 |
0.111 |
0.158 |
0.008 |
0.005 |
Analysis 4 |
59.018 |
38.198 |
2.210 |
0.206 |
0.126 |
0.231 |
0.007 |
0.004 |
Analysis 13 |
60.353 |
37.837 |
1.328 |
0.207 |
0.099 |
0.167 |
0.005 |
0.004 |
Analysis 25 |
58.441 |
37.342 |
3.756 |
0.192 |
0.122 |
0.129 |
0.012 |
0.006 |
Conclusion
The accuracy of Micro-XRF analysis with XTrace is established for the copper alloy ERM®-EB375 (CuZn39Pb3). Results for the elements Mn, Co, Ni, and Sn indicate only a small deviance from the certified values, which is adequate for the majority of applications. Even though there is a large variation in the Pb content of the single analyses, the average value is close to the certified value. Co and Mn were only detected by using Micro-XRF. This was because the concentrations were below the LOD of the EDS method, which is 1000 ppm (0.1 mass%).
Primary filters were a great help in suppressing the diffraction reflexes in X-ray fluorescence spectra and allowed the intensity of elements whose peaks would otherwise be overlayed by diffraction peaks to be determined.
The new ESPRIT 2 software function Image extension was very supportive in analyzing the heterogeneous copper alloy samples by allowing the acquisition of different point spectra over a large surface area.
The low detection limit of Micro-XRF with high spatial resolution of electron excitation demonstrated a powerful combination of methods for alloy characterization.
Acknowledgements
The author would like to thank V.D. Hodoroaba from BAM (Federal Institute for Materials research and Testing, Berlin) for providing the sample and M. Procop from IfG (Institute Scientific Instruments GmbH, Berlin).
Author
Birgit Hansen, Application Scientist EDS & Micro-XRF/SEM, Bruker Nano GmbH, Berlin, Germany
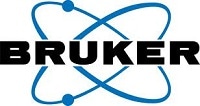
This information has been sourced, reviewed and adapted from materials provided by Bruker Nano Analytics.
For more information on this source, please visit Bruker Nano Analytics.