The need for development of smaller, more efficient energy storage materials is ever increasing in order to support the widespread adoption of portable electronic devices such as tablets and smart phones. In 1973, the concept of using lithium ion batteries was initially proposed, where Li+ ions act as charge carriers that move towards the positive electrode during use and then change direction during charging. Since the commercialization of this technology in 1991, it has superseded nickel hydride as the battery material for use in consumer electronics applications. In recent times, Li ion batteries are being used as an alternative to lead-acid batteries for bulkier vehicle and aerospace applications.
Classical lithium ion batteries are based on a liquid electrolyte but significant developments have been made towards using solid state thin-films as a replacement to liquid electrolytes. Thanks to their higher average output voltage, longer cycling life, lighter weights and as a result higher energy density, thin-film lithium ion batteries provide better performance than typical liquid electrolyte batteries. To build a thin film battery, all battery components, including an anode, a cathode, current leads and a solid electrolyte, need to be fabricated into multi-layered thin film. Solid state micro-batteries widely use lithium phosphorous oxynitride (LiPON) owing to ease of preparation, increased durability to cycling and low electronic conductivity. Although LiPON is widely used, only little is known regarding Li mobility and nitrogen bonding.
This article discusses the investigation of bulk and surface chemistry of LiPON films formed via atomic-layer deposition (ALD) using conventional surface analysis techniques of XPS and sputter depth profiling. XPS provides quantitative data on the elemental composition of the near surface region to a depth of <10 nm. The elemental composition is studied as a function of depth and the results collected from conventional monatomic depth profiling are then compared with the results from cluster depth profiling.
Experimental
The AXIS photoelectron spectrometer equipped with a gas-cluster ion source (GCIS) was used to perform all measurements. The GCIS was run in unique high-energy cluster mode for these experiments, using 20 kV Ar1000+. Depth profiling was performed in a practical time frame using high-energy cluster ions. The effects of layer blending and sputter roughening were reduced by using sample rotation throughout the profiles. The ALD method was used to produce LiPON thin-films using Li precursors and a N2 plasma1. LiPON films were previously analyzed by optical ellipsometry to be ~50 nm thick.
Results and Discussion
After introduction into the analysis chamber a survey spectrum was acquired from the as-received sample. The presence of the constituent elements Li, P, O and N was confirmed by the analysis of the survey spectrum. The analysis also showed a significant amount of carbon, which could be due to the presence of adsorbed species from air exposure. Depth profiling was carried out to study Li distribution throughout the film. Figure 2 compares traditional monatomic depth profiling using 4 kV Ar+ ions and the same sample profiled with 20 kV Ar1000+ ions.
.jpg)
.jpg)
Figure 1. Depth profile of 50 nm LiPON thin-film using (a) 5 kV Ar+ (b) 20 kV Ar1000+.
Both depth profiles were conducted as an overnight experiment using the cluster mode etch rate >4 nm/minutes. A distinct difference can be observed between the two profiles. Under monatomic bombardment, Li concentration is initially increased after the first etch cycle due to removal of surface contamination and then subsequently decreased in the bulk of the film reaching a steady-state of ~25.5 atomic%. Near the LiPON interface with Si substrate, there is a significant increase in Li concentration, reaching a maximum of 44%.
.jpg)
Figure 2. Li ion concentration comparison between monatomic (black) and cluster profile (red).
Conversely, a different Li distribution is observed for the profile obtained when profiled with 20 kV Ar1000+ ions. Li concentration is initially increased after the first etch but no subsequent decrease in Li concentration is observed after reaching a steady state of >31%. This translates to an increase of 22% Li across the film depth in comparison with the monatomic profile. Interestingly, accumulation of Li was not observed at the interface with the Si substrate. Figure 3 shows a comparison of the Li concentrations profiled using two different ions.
.jpg)
Figure 3. Schematic of ion action on light elements.
Earlier, monatomic Ar+ bombardment of glasses has demonstrated lower than expected concentrations of light elements such as sodium2 due to accumulation of positive charge in the near-surface region from implanted Ar+ ions. The small, mobile positive ions in the surface are repelled further by the charge build-up into the bulk, causing an underestimation in surface quantification. The same mechanism of Li ion repulsion and bulk migration is proposed to take place for LiPON thin-films analyzed here. Li migration takes place under monatomic profiling, leading to an underestimation of Li concentration. Repulsive bulk migration also plays a role in the increase of Li concentration at the interface with the Si substrate. The light ions accumulate at the interface as they cannot penetrate through into the native silicon oxide. It is important to note that this system is especially susceptible to this process owing to the fact that by their design, the Li+ ions in LiPON are mobile for the charge transfer process.
Conclusion
It has been shown that quantitative information regarding the composition of LiPON thin-films produced via ALD can be obtained using the combination of XPS and depth profiling techniques. It has been demonstrated that monatomic Ar+ ions are unsuitable for use in profiling of materials with mobile, light elements as the accumulation of positive charge can cause migration, which, in turn, results in incorrect stoichiometry. In conclusion, the use of high energy Arn+ clusters for depth profiling, this class of materials is vital to mitigate the effects of ion migration and gain confidence in the validity of the results.
Acknowledgments
Many thanks to the research group of Prof. Gary Rubloff and in particular Alex Pearse at the University of Maryland.
References and Further Reading
- A. Kozen, A. Pearse, G. Rubloff, C-F Lin, M. Noked, Chem. Mater., 2015, 27, 5324–5331
- Y. Yamamoto, K. Yamamoto, J. Non. Cry. Sol., 2015, 356, 14
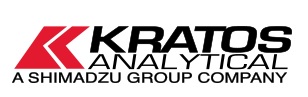
This information has been sourced, reviewed and adapted from materials provided by Kratos Analytical, Ltd.
For more information on this source, please visit Kratos Analytical, Ltd.