This article describes the test results of a blood bag sample performed by Jordi Labs for one of its clients. The tests performed are as follows:
- Scanning Electron Microscopy with Energy Dispersive X-Ray Spectroscopy (SEM-EDX)
- Fourier Transform Infrared Spectroscopy Microscopy (FTIR-Micro)
- Pyrolysis Mass Spectrometry (PYMS)
Objective
Thin films which are used in packaging can be far more complex than their simple appearance may suggest. Such samples may include multiple layers, which sometimes measure just a few microns thick, so as to increase the favorable properties that can be obtained from the varied chemistries of each layer.
In this study, analysis of the inside and outside of the blood bag revealed two different chemistries on the bag. Additional studies revealed the presence of more layers, all of which may play critical roles in the sample’s final properties. The aim of this case study was to analyze the blood bag through multiple methods so that the various layers present in the sample can be identified and measured.
Summary of Results
Table 1 shows a summary of the layers of the blood bag, and Figure 1 shows a cross-section. Four separate polymeric layers were observed in the blood bag sample: two polyamide layers and two polyethylene layers; one of the layers contained a secondary polymer, for example polyvinyl alcohol.
Using FTIR-micro, a cross-section of the blood bag was mapped and the bulk chemistry of each layer was identified. Even the differences between the two polyamide layers were clearly distinguished. More accurate thickness information on each film layer was obtained using SEM-EDX. This technique could also be used to identify which layers contained considerably more O, N and C.
Selective dissolution was finally applied to effectively separate the four layers into extra films. These films were subsequently analyzed by PYMS to validate the identifications made by FTIR-micro and offer more definitive polymer identifications beyond bulk chemistry. PYMS was also able to detect a mixture of polyamides present in the fourth layer, which could not be distinguished from each other with FTIR-micro alone.
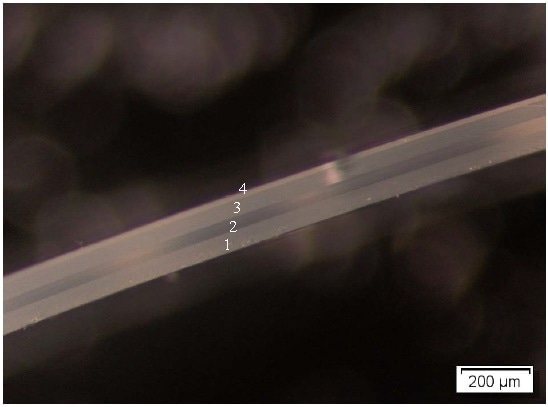
Figure 1. Cross-section of blood bag with numbered layers.
Table 1. Summary of Analysis for Blood Bag.
Layer Number |
Approximate Layer Thickness by SEM (μm) |
FTIR Identification |
Layer Major Elements by EDX |
Solvent In Which Layer was Insoluble |
PYMS Identification |
1 |
39 |
Polyethylene |
C |
HFIP |
Polyethylene |
2 |
39 |
Polyamide/PVA |
C, N, O |
TCB |
Nylon 6/PVA |
3 |
41.5 |
Polyethylene |
C |
HFIP |
Polyethylene |
4 |
11.6 |
Polyamide |
C, N |
TCB |
Nylon 6,6/Nylon 6 |
Initial Investigation
Figure 2 shows the blood bag sample, which was first tested using attenuated total reflectance mode FTIR analysis. Yet, it was observed that the outside and inside surfaces produced considerably different FTIR spectra, as shown in Figure 3. This prompted additional investigation into the composition of the bag, analyzing the chemical composition and number of the layers.
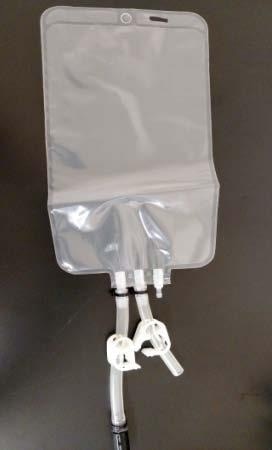
Figure 2. Blood bag sample.
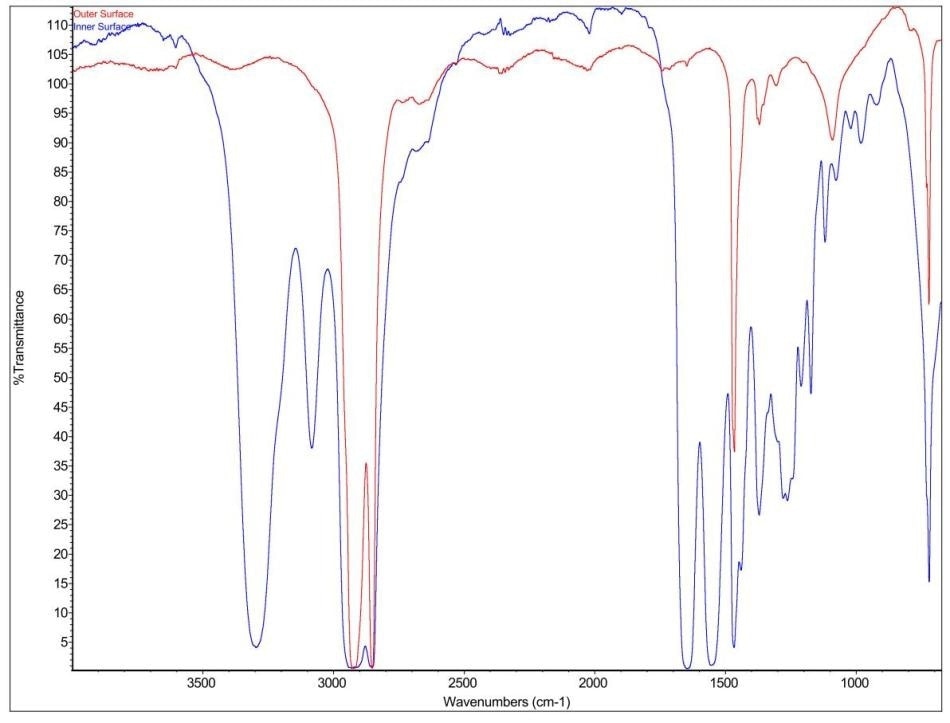
Figure 3. FTIR spectra of inner (blue) and outer (red) layers of the blood bag.
Please click here if you would like more information on the product in this article or a quote
Sample Preparation and Optical Microscopy
A scalpel was used to cross-section the sample and this was imaged by optical microscopy. As shown in Figure 4, the blood bag was found to contain four layers that were visible by optical microscopy. These layers were sufficiently large and hence could be measured; this is summarized in Table 2. However, Scanning Electron Microscopy is recommended for smaller layers for better accuracy, as shown in Figure 12.
Table 2. Layer Thickness by Optical Microscopy.
Layer |
Thickness |
1 |
54.56 |
2 |
37.34 |
3 |
47.79 |
4 |
15.28 |
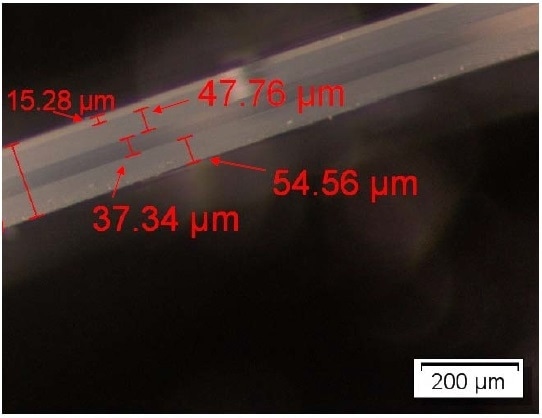
Figure 4. The cross-sectioned blood bag with layer measurements.
FTIR-Microscopy
Within a diamond cell, a wedge-shaped cross-sectional piece of the sample was cut and compressed resulting in a suitable transparent sample for mapping with transmission mode FTIR, as illustrated in Figure 5. This sample was subsequently examined with FTIR transmission mode line mapping, the map of which is shown in Figure 6.
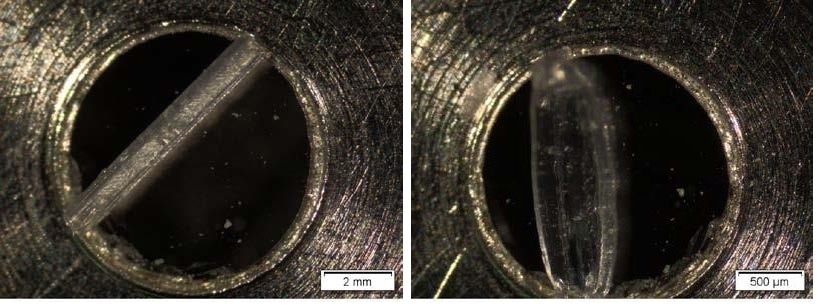
Figure 5. Cross-sectional wedges of blood bag on a diamond cell before (left) and after (right) compression.
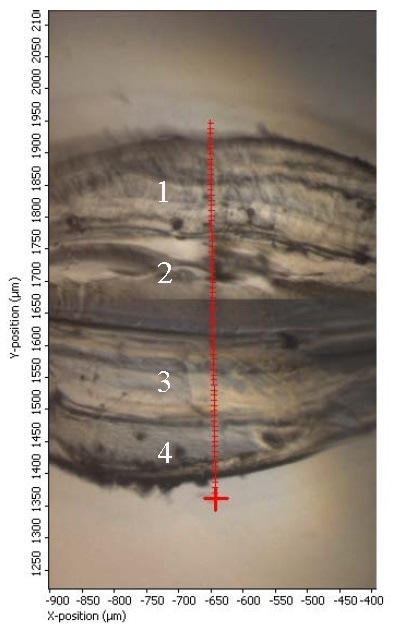
Figure 6. FTIR micrograph of blood bag mapped area (dotted red line) with numbered layers
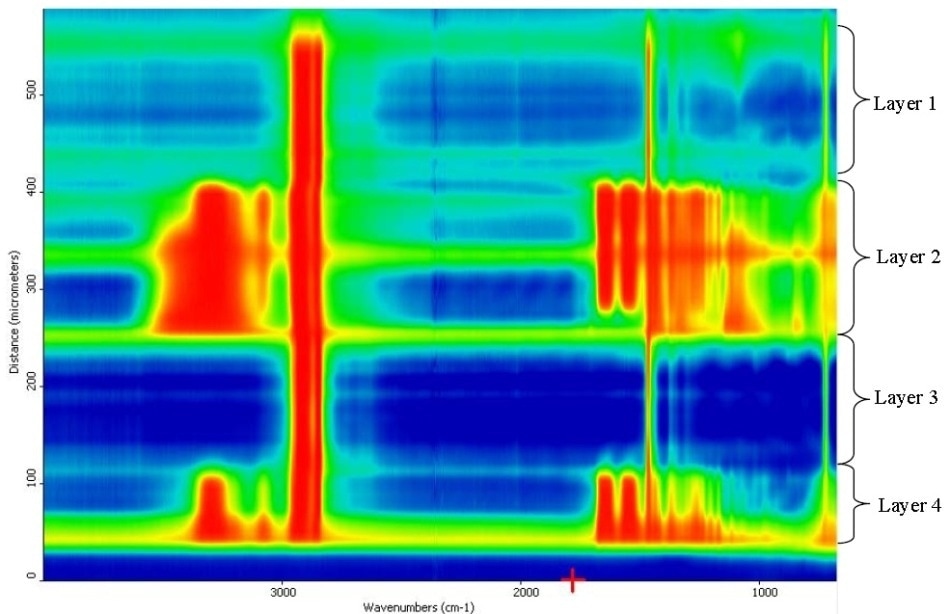
Figure 7. 2D FTIR line map of blood bag. Blue represents weak transmittance while red represents strong transmittance.
Figure 7 shows the 2D FTIR line map, where along the line map (red dotted line in Figure 6) the intensities of the spectra change for each wave number. Besides the FTIR micrograph, this demonstrates that the FTIR spectrum is varying from one layer to another. Four distinct regions with continuous spectra can be viewed which are consistent with the four layers detected visibly by optical microscopy. The layers have been observed on the right side of Figure 7.
Next, FTIR spectra were chosen from each region, which are illustrated in a stacked overlay in Figure 8. The stacked overlay reveals that similar spectra are shown by layers 1 and 3 as well as by layers 2 and 4. Table 3 lists potential functional group identifications based on wavenumber for all the samples.
It was observed that layers 1 and 3 are consistent with polyethylene, while layers 2 and 4 had a number of peaks in common with a polyamide, for example Nylon. The spectra for layers 2 and 4, however, showed some major differences in peak shape as well as the fingerprint region of the FTIR spectra. This indicates that the two layers are related to polyamide but one could be modified or include a co-polymer. Layer 2, for instance, contains a stronger peak at 1100 cm-1 and a broader peak around 3300 cm-1. As a result, some important similarities are observed when comparing the layer 2 spectrum with polyvinyl alcohol (PVA), illustrated in Figure 9. By contrast, layer 4 shows a very clear match to polyamide, with no major peaks unaccounted for, as shown in Figure 10.
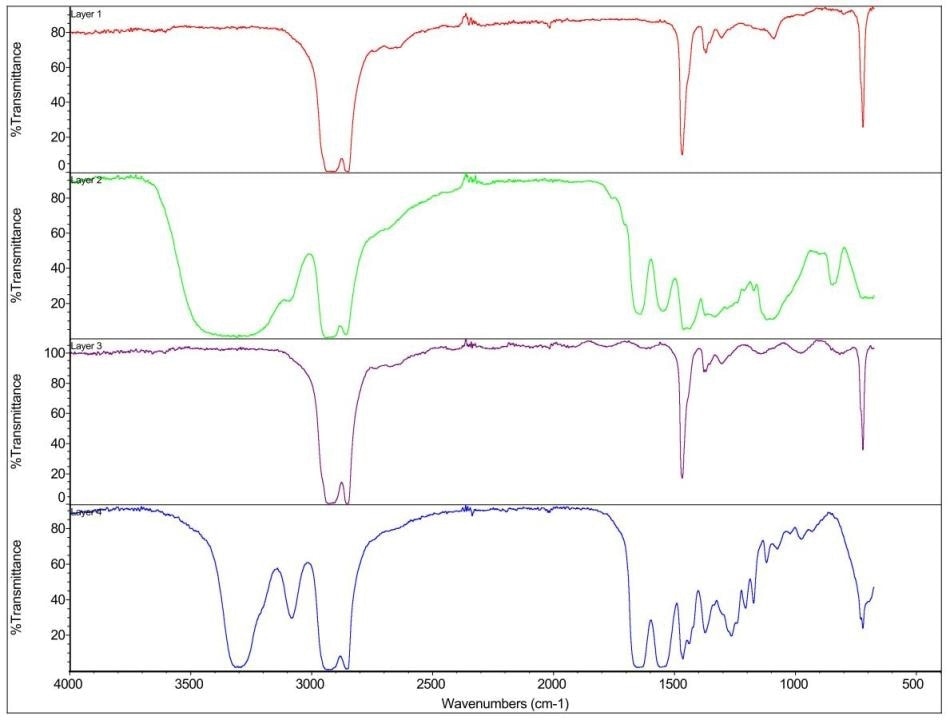
Figure 8. Stacked FTIR spectra of blood bag layer 1 (red), layer 2 (green), layer 3 (purple), and layer 4 (blue).
Table 3. FTIR Peaks and Identifications of Blood Bag Layers.
IR Frequency (cm-1) |
Functional Group |
Layer 1 |
Layer 2 |
Layer 3 |
Layer 4 |
- |
3308, 3090 |
- |
3312, 3080 |
NH stretch |
2917, 2850 |
2935, 2858 |
2930, 2850 |
2924, 2856 |
CH stretch—aliphatic |
- |
1641 |
- |
1653 |
C=O stretch |
- |
1544 |
- |
1558 |
N-C=O stretch |
1467 |
1462 |
1467 |
1464 |
CH2 bend |
1369 |
1331 |
1369 |
1372 |
CH bend |
- |
1116 |
- |
1263, 1118 |
CN stretch |
1088 |
844 |
- |
1073 |
C-O stretch |
719 |
719 |
719 |
720 |
CH2 rock |
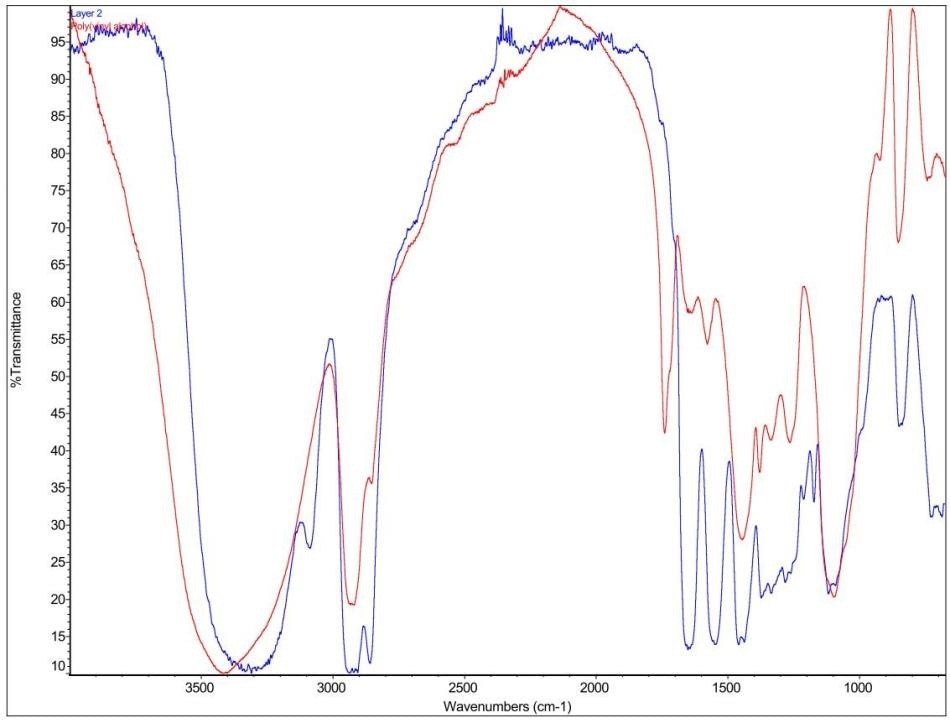
Figure 9. FTIR spectra of blood bag layer 2 (blue) and PVA (red).
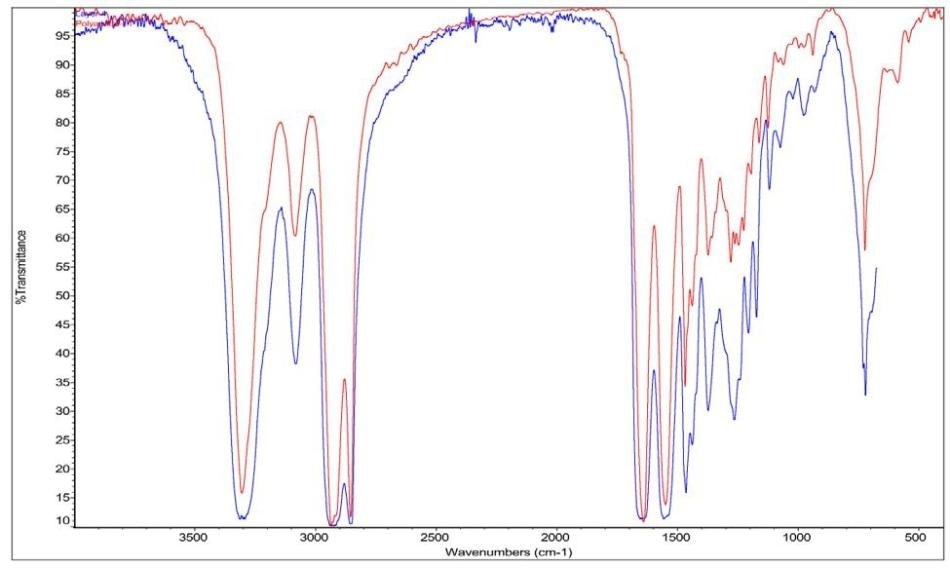
Figure 10. FTIR spectra of blood bag layer 4 (blue) and polyamide (red).
SEM/EDX
The backscattered electron (BSE) and secondary electron (SE) images of the samples are shown in Figure 11. These images were then measured, as shown in Figure 12, in order to acquire another measurement thickness for each layer. Compared to the optical microscope imaging in Figure 4, the values are slightly different demonstrating how SEM offers a higher level of accuracy when it comes to film measurements.
Next, the sample was analyzed by EDX mapping, looking for the distribution of oxygen, nitrogen, and carbon in the sample. Figure 13 shows the EDX elemental maps for the blood bag. As can be seen, layer 2 contains considerable amounts of oxygen, while layers 2 and 4 contain considerably higher amounts of nitrogen. This is in agreement with the FTIR analysis, which found those layers to be consistent with polyamides and layer 2 to contain another component consistent with EVA. Although EDX analysis is often useful for discerning inorganic species and is only a semi-quantitative method, the minor differences in nitrogen and oxygen content can be observed and applied to support the FTIR analysis.
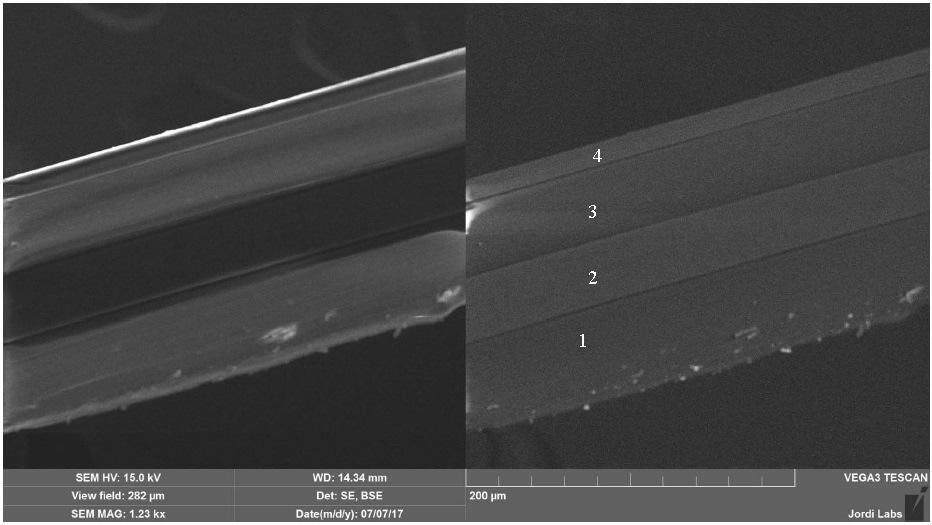
Figure 11. SEM SE (left) and BSE (right) images of blood bag, with numbered layers.
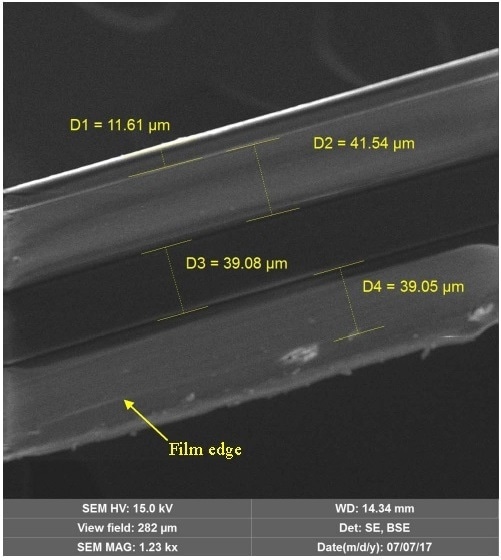
Figure 12. Measured SEM SE image of blood bag.
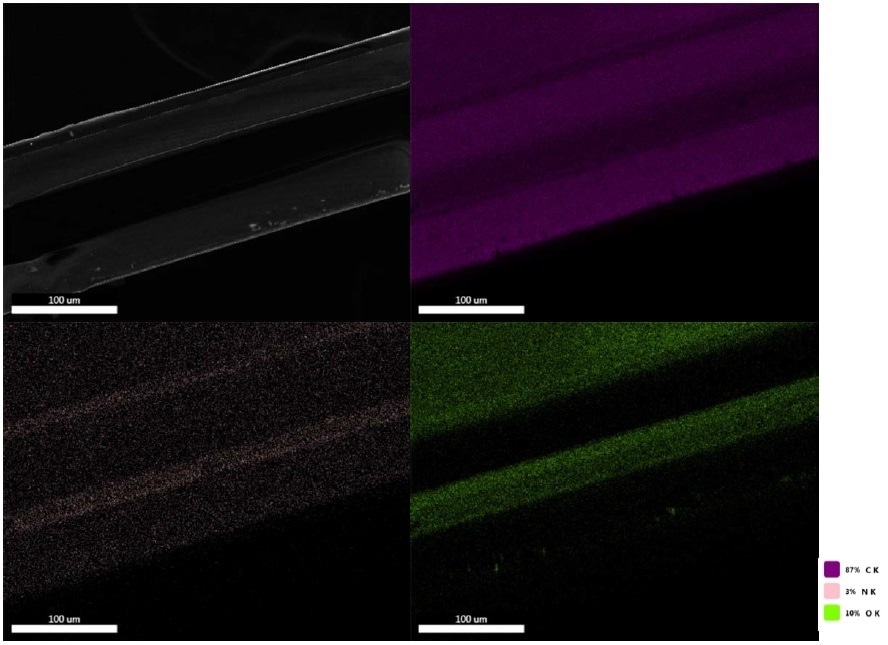
Figure 13. Individual EDX Element Maps for blood bag, showing the mapped area with SEM imaging (top left), carbon map (purple, top right), nitrogen map (pink, bottom left), and oxygen (green, bottom right).
Pyrolysis Mass Spectrometry (PYMS)
While the film’s bulk chemistry has been further revealed by SEM-EDX and FTIR, PYMS carried out on the individual layers can give a more definitive identification of the polymer layers. Separation was achieved by immersing part of the blood bag sample in HFIP. The film partly dissolved, but two smaller films remained which were separately tested by PYMS.
A double shot technique was used to perform the analysis by PYMS. In the double shot experiment, a sample is heated to release volatiles and these were cryogenically trapped and tested by GCMS. After 1st pass analysis was completed, the remaining part of the sample was quickly heated above the decomposition temperature, and pyrolyzed components were allowed to pass into a gas chromatography column which were then analyzed by mass spectrometry. Prominent peaks were detected in PYMS that mostly included polymer fragments as well as fragments of antioxidants, monomer and other additives. The NIST/EPA/NIH mass spectral search program was used to compare the sample peaks with more than 796,613 reference compounds.
During the first pass, no prominent peaks were observed for the HFIP-insoluble films, but the second pass generated two chromatograms as depicted in Figure 14. Based on the chromatogram peaks, both films were observed to be consistent with one another, and the fragments observed were found to be consistent with polyethylene. This agrees with the findings by FTIR, which observed layers 1 and 3 to be consistent with polyethylene, and either of these would not be expected to dissolve in HFIP.
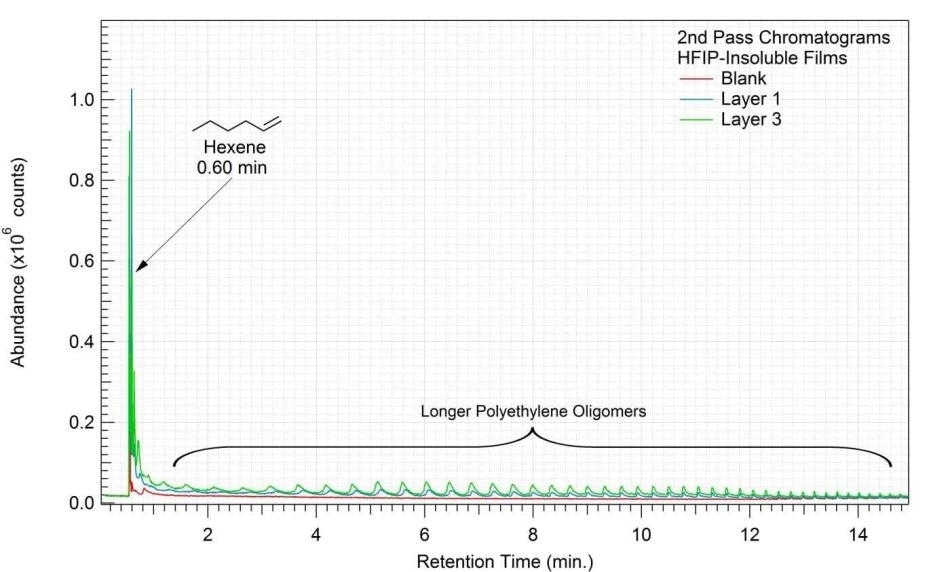
Figure 14. PYMS Chromatograms of HFIP-Insoluble layers from blood bag.
In order to separate the other layers, the polyethylene layers were dissolved by placing a new portion of the blood bag film in hot TCB. Once again, the film partly dissolved but two smaller films remained, which were separated easily. PYMS was used to separately test these films.
During the first pyrolysis pass, no prominent peaks were observed for the TCB-insoluble films but the second pass generated two chromatograms, as illustrated in Figure 15. The peaks in the two films were found to be consistent with polycaprolactam, which is also called Nylon 6.
However, degradants related to PVA were detected in one of the films but these were absent from the other polyamide film. In addition, unique fragments related to the polyamide Nylon 6,6 were present in the film without PVA fragments. This is consistent with the FTIR results, which detected both layers 2 and 4 being related to polyamide, but with layer 2 comprising of another co-polymer, such as PVA. FTIR-micro will not be able to distinguish that both Nylon 6,6 and Nylon 6 were present in layer 4; however, the two polymer species can be determined by PYMS.
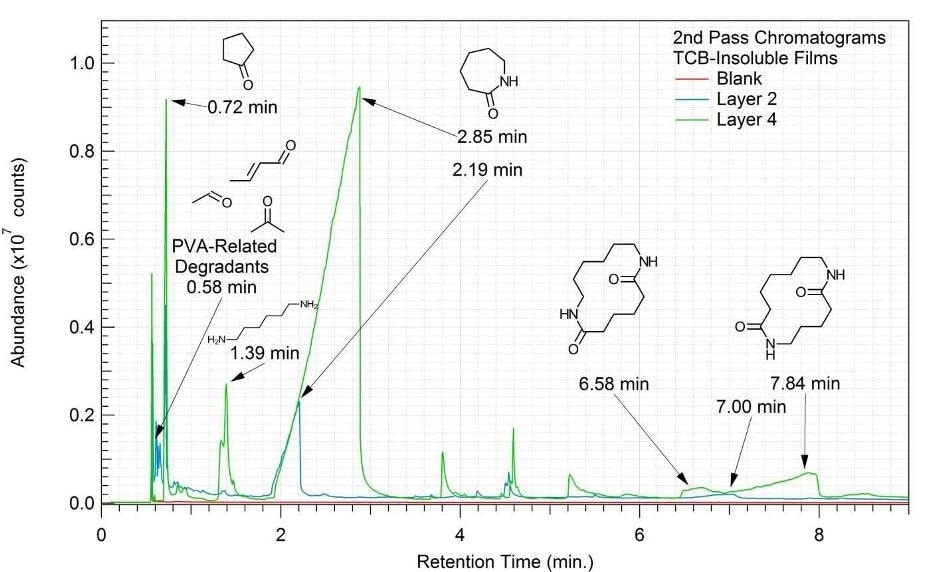
Figure 15. PYMS Chromatograms of TCB-Insoluble layers from blood bag.
Analysis Conditions
This section of a Jordi Labs report provides data about the types of methods used including sample preparation, instrument type, solvents, temperatures and so on. The specific conditions have been removed for this case study.
Closing Comments
Jordi Labs’ reports are mainly issued for the use of the clients to whom they are addressed. No use of the Jordi name or quotations from reports is permitted except as authorized in writing. The liability of Jordi Labs with regards to the services provided shall be restricted to the amount of consideration paid for such services and do not include any significant damages.
Jordi Labs deals in polymer testing and has three decades of experience in complete polymer deformulations. It is one of the few laboratories in the country that specializes in this type of testing. Jordi Labs can work closely with its clients to help explain their test results and solve their problems.
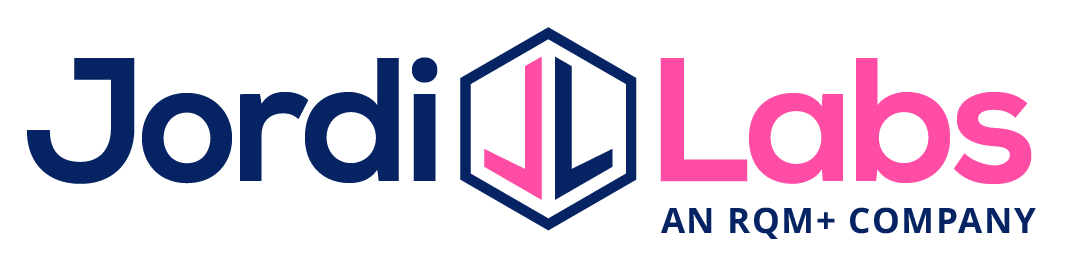
This information has been sourced, reviewed and adapted from materials provided by Jordi Labs.
For more information on this source, please visit Jordi Labs.