This article focuses on the application of thermogravimetric analysis (TGA), thermomechanical analysis (TMA), and dynamic mechanical analysis (DMA) techniques. It comprehensively discusses the various effects such as expansion, decomposition, glass transition, cold crystallization, relaxation, melting, and recrystallization. The TGA, DMA, and TMA techniques provide useful complementary information to DSC measurements.
Thermogravimetric Analysis (TGA)
The TGA technique determines the mass of a sample while it is cooled, heated, or maintained isothermally in a defined atmosphere. This technique is primarily used to quantitatively analyze products.
A normal TGA curve reveals the mass loss steps pertaining to the loss of volatile components (monomers, solvents, moisture), combustion of carbon black, polymer decomposition, and final residues (filler, ash, glass fibers). The technique enables one to examine the decomposition of materials and products and to draw conclusions regarding their separate components.
The DTG curve is the first derivative of the TGA curve with regards to time. It is proportional to the rate of sample decomposition. In a DSC/TGA measurement, DSC signals as well as weight information are recorded at the same time. This makes it possible to detect and evaluate exothermic or endothermic effects. However, the DSC signal, which is recorded in DSC/TGA measurement, is less sensitive than that acquired from a dedicated DSC instrument. In addition, the DSC curves are less well resolved.
In Figure 1, the upper diagram illustrates DTG and TGA curves of PET, while the two lower diagrams are the corresponding DSC curves quantified in a nitrogen atmosphere. On the right side, the DSC curve in the range up to 300 °C reveals the cold crystallization, glass transition, and the melting process.
It is possible to correct the DSC signal for the mass lost by the sample at the time of measurement (left); the red and blue curves are the corrected and uncorrected curves for the loss of mass2, 3, respectively.
.jpg)
Figure 1. Measurement curves of PET recorded from 30 to 1000 °C at a heating rate of 20 K/minute using a TGA/DSC 1 equipped with a DSC sensor. The TGA curve shows the change in mass of the sample and the DSC curve the endothermic or exothermic effects.
Decomposition
Chemical bonds break during a decomposition process, and complex organic polymers or compounds decompose to form gaseous products, for example, water, hydrocarbons, or carbon dioxide.
Under inert or non-oxidizing conditions, organic molecules may also decompose and form carbon black. It is possible to detect volatile decomposition products by joining the TGA to a mass spectrometer (MS) or Fourier transform infrared spectrometer (FTIR).
Thermomechanical Analysis (TMA)
TMA measures a sample’s dimensional changes as it is cooled or heated in a defined atmosphere. A normal TMA curve exhibits expansion, which is below the glass transition temperature, the glass transition (observed as a change in the slope of the curve), expansion above the glass transition temperature, and plastic deformation.
Measurements can be carried out in the penetration mode, the dilatometry mode, or the Dynamic Load TMA (DLTMA) mode.
Dilatometry
Dilatometry involves the measurement of the expansion or shrinkage of a sample. Due to this reason, the force used is considerably low and is just enough to guarantee that the probe stays in contact with the sample. The measurement result is the coefficient of thermal expansion (CTE). The dilatometry measurement illustrated in Figure 2 was carried out using a sample with a thickness of about 0.5 mm sandwiched between two silica disks. First, it was preheated to 90 °C in the instrument to remove its thermal history. Upon cooling, it was measured in the range of 30–310 °C at a heating rate of 20 K/minute with the help of the ball-point probe at a very low force of 0.005 N.
.jpg)
Figure 2. TMA measurement of PET in the dilatometry mode
In Figure 2, the curve in the upper diagram demonstrates that the sample expands only gradually up to the glass transition. Then, the expansion rate considerably increases upon further heating as a result of the increased mobility of the molecules in the liquid state. Subsequently, the sample shrinks as a result of cold crystallization and recrystallization processes. Again, the sample expands following the formation of crystallites above the temperature of nearly 150 °C, and it melts eventually. As part of the melting, there is a drastic reduction in sample height and viscosity.
Penetration
During the penetration measurements, information related to temperatures are mainly obtained. In general, the sample thickness is not so significant since the contact area of the probe with the sample varies at the time of the experiment. The penetration depth is impacted by the sample geometry as well as the force used for the measurement.
To measure the penetration, a sample with a thickness of about 0.5 mm was placed on a silica disk and the ball-point probe was made to rest directly on the sample. The measurements were carried out in the range of 30 to 300 °C at a heating rate of 20 K/minute at forces of 0.1 and 0.5 N. Here, the sample was not preheated.
While performing the penetration measurement, the probe penetrates increasingly into the sample. The ordinate signal considerably decreases at the glass transition, it stays almost constant following cold crystallization, and subsequently decreases again upon melting (Figure 3).
.jpg)
Figure 3. TMA of PET measured in the penetration mode
DLTMA
Dynamic Load TMA (DLTMA) is a highly sensitive technique for determining physical properties. Contrary to DSC, it involves characterizing the mechanical behavior of samples. In DLTMA4, a low force and a high force alternately act on the sample at a specified frequency. This enables the measurement of the expansion, weak transitions, and the elasticity (Young’s modulus) of samples. The stiffness of the sample is inversely proportional to the amplitude.
In Figure 4, the measurement curve illustrates the glass transition at 72 °C, after which the material expands in the liquid state; since the material is soft, the amplitude is large. After this, cold crystallization occurs, where the PET shrinks and there is a reduction in the amplitude. At a temperature of 140 °C, the sample once again becomes hard. Then, the sample expands when the temperature further increases to 160 °C.
.jpg)
Figure 4. DLTMA measurement of PET from room temperature to 160 °C.
Dynamic Mechanical Analysis (DMA)
Dynamic mechanical analysis involves the measurement of the mechanical properties of a viscoelastic material as a function of temperature, time, or frequency when the material is subjected to a periodically oscillating force.
A typical measurement involves the application of an oscillating force to the sample at distinctive frequencies. The elastic modulus is measured as the shear storage modulus, G′, and loss modulus, G″. Tan delta, the loss factor, or the damping coefficient, G″/G′, is calculated by using this data. When compared to other methods, DMA is considerably more sensitive. For instance, it has the ability to measure glass transitions of thin layers or filled materials on a substrate material, that is, transitions which are challenging to be detected using DSC.
The DMA measurement curve of a shock-cooled PET sample with a diameter of 5 mm and a thickness of 0.49 mm in the shear mode at 1 Hz in the temperature range of –150 to +270 °C is illustrated in Figure 5. The heating rate was 2 K/minute.
.jpg)
Figure 5. DMA shear measurement of PET in the range of –150 to +270 °C.
Apart from the effects detected by TGA/DSC or TMA (for example, crystallization, glass transition, and melting), the DMA curve also illustrates other effects such as β relaxation (local movement of polymer groups) or recrystallization. Since β relaxation is weak, it can be measured only by using DMA. This transition cannot be detected by other thermal analysis techniques, such as TGA or DSC.
Overview of the Effects and Comparison of the Results
Figure 6 illustrates the different thermal techniques adopted for the analysis of PET. In Table 1, the effects that can be measured by different thermal techniques have been summarized. In Table 2, the results acquired for PET with the help of the different techniques are compared. The temperatures specified for DSC and TGA/DSC relate to peak temperatures, the TMA temperatures to the start of the variation in expansion, and the DMA temperatures to the peaks in the tan delta curve.
.jpg)
Figure 6. Overview of the effects and comparison of the results
Table 1. Effects measured by different analytical methods
Effects |
DSC |
TGA/DSC |
TMA |
DMA |
β relaxation |
|
|
|
x |
Glass transition |
x |
x (DSC signal) |
x |
x |
Cold crystallization |
x |
x (DSC signal) |
x |
x |
Recrystallization |
(x) |
|
|
x |
Melting |
x |
x |
x |
x |
Decomposition |
(x) |
x |
(x) |
|
OIT |
x |
|
|
|
Table 2. Comparison of the results of PET determined by different techniques
Effects |
DSC
(20 K/min) |
TGA/DSC
(20 K/min, DSC, N2) |
TMA
(20 K/min) |
DMA
(1 Hz, 2 K/min, tan delta) |
β relaxation |
|
|
|
–77 °C |
Glass transition |
80 °C |
81 °C |
77 °C |
81 °C |
Cold crystallization |
150 °C |
154 °C |
152 °C |
118 °C |
Recrystallization |
|
|
|
183 °C |
Melting |
248 °C |
251 °C |
242 °C |
254 °C |
Decomposition |
|
433 °C |
|
|
It is clear that the different techniques complement one another, provide consistent results, and offer vital information for characterizing material properties. This is specifically helpful for the investigation of unknown materials, in the quality control of substances, or in the analysis of failure and damage, for instance, for the detection of probable impurities in a material. Practically, a comprehensive analysis employing different techniques is highly informative.
Conclusions
This article discussed the various possibilities available for the characterization of a thermoplastic using thermal analysis. TMA, DSC, DMA, and TGA were the methods adopted. PET was the thermoplastic selected for the measurements. The results are in good agreement with one another. Glass transition, melting, cold crystallization, decomposition, and recrystallization were the main effects examined. Topics such as the thermal history of samples and OIT were also covered. Effects similar to those detailed for PET occur with other polymers. Usually, it is possible to measure a specific effect by different thermal analysis methods. The results acquired with the help of one method are used to confirm those from another method. For the comprehensive characterization of materials, in general, the samples are first examined using TGA, then using TMA and DSC, and finally using DMA.
References and Further Reading
- A. Hammer, Thermal analysis of polymers. Part 1: DSC of thermoplastics, UserCom 31, 1–6.
- R. Riesen, Heat capacity determination at high temperatures by TGA/DSC. Part 1: DSC standard procedures, UserCom 27, 1–4.
- R. Riesen, Heat capacity determination at high temperatures by TGA/DSC. Part 2: Applications, UserCom 28, 1–4.
- PET, Physical curing by dynamic load TMA, UserCom 5, 15.
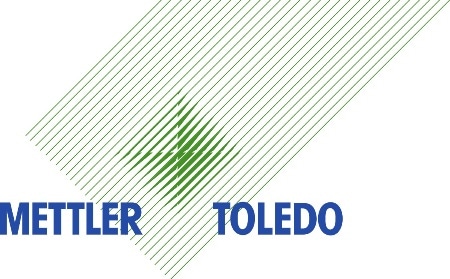
This information has been sourced, reviewed and adapted from materials provided by Mettler Toledo - Thermal Analysis.
For more information on this source, please visit Mettler Toledo - Thermal Analysis.