Both surface plasmon resonance (SPR) spectroscopy and its counterpart, localized surface plasmon resonance (LSPR) spectroscopy, have been accepted as important means for carrying out not only nanostructure characterization but also label-free chemical and biological sensing.
SPR spectroscopy is mostly used in biosensing, especially when investigating binding affinities, such as antibody-antigen interactions. In contrast, LSPR spectroscopy is often used as a means of signal enhancement in the detection of trace molecules.
LSPR has been identified as the physical phenomena behind methods including:
- Surface-enhanced Raman spectroscopy
- Infrared spectroscopy
- Absorption spectroscopy
- Fluorescence spectroscopy
Firstly, this article will investigate the underlying physics of surface plasmons, including their wavelength-dependent interactions.
Secondly, it will contrast the differences between SPR and LSPR, going on to scrutinize the processes involved in signal enhancement.
Thirdly, two short case studies will be reviewed in order to explore applications of SPR and LSPR spectroscopy.
Surface Plasmon Physics
Surface waves generated from the coherent oscillation of conduction band electrons, along with the interface between a dielectric and metal, will result in what is known as surface plasmons.
Surface plasmons, like other electromagnetic waves, have an associated wavevector whose magnitude is determined by the relative permittivity (also referred to as the dielectric constant) of the propagating media.
Relative permittivity is equal to the square root of the index of refraction, and this is the case with non-magnetic materials. Consequently, it can be said that relative permittivity has a similar wavelength dependence as the index of refraction.
As a result of this dependency, when the parallel component of the incident light’s wavevector is in resonance with the wavevector of the surface plasmon, it will induce either wavelength-dependent constructive or destructive interference of the two waves.
Depending on the conditions, both LSPR and SPR may result in either a wavelength increase or decrease, in the reflected or transmitted spectrum.
SPR sensors are usually constructed from a thin metal film - often silver or gold – applied to a dielectric material such as glass. Broadband light is directed at the interface by means of total internal reflection (TIR). SPR sensors produced in this manner rely on prisms to ensure TIR, similar to an ATR (attenuated total reflection) tip in FT-IR.
Other approaches may utilize TIR within coated optical fibers to induce SPR. In SPR spectroscopy, the sensor is exposed to an analyte, resulting in a bond to the sensor. This can lead to a slight change to the relative permittivity and subsequently changes in the resistance frequency of the surface plasmons.
In SPR, when the incident light is in residence with the surface plasmons, the redetect spectra will exhibit a dip in the spectrum which corresponds to destructive interference.
As a result, the local minima of the transmission spectrum will shift as the sensor collects increasing amounts of analyte. This can facilitate very sensitive quantitation.
Gauss’s Law states that the surface charge density of a conductor is inversely proportional to its radius. In the case of LSPR, nanofabrication techniques are utilized to make use of this localization effect.
A description of the underlying physics is outside of the scope of this article, but it is important to understand that this resultant extremely high surface charge density could lead to substantial signal enhancement.
This enhancement only takes place when the real part of the substrate’s relative permittivity is a negative multiple of that of the environment. This can increase the strength of the signal.
Gold, with its relative permittivity of -22.855 + 1.4245 i at 785 nm, and silver, with its relative permittivity of -11.755 + 0.37038 i, are commonly used in SPR and LSPR for this reason.
In surface-enhanced Raman spectroscopy (SERS), gold is typically used for 785 nm excitation while silver is commonly used for 532 nm excitation.
The magnitude of the scaling factor referred to earlier is dependent on the geometry of the substrate, which can range from between two to twenty. The ‘enhancement’ wavelength can be tuned depending on the shape of the nanostructure. This geometric dependency is beneficial when characterizing nanomaterials.
Fiber Optic SPR Probes
The incorporation of SPR sensors into fiber-optic probes means targeted sensors can be deployed in hazardous environments.
Typically, probes are manufactured by first removing the cladding from a section of fiber optic cable. Then, the section is coated with a metallic layer, followed by a dialectic layer.
The Indian Institute of Technology Delhi followed this process, and here, fiber core was coated with a layer of silver, then a layer of zinc oxide, in order to detect chlorine gas.1
In this instance, a broadband light was integrated into the probe using an Avantes AvaLight-HAL, tungsten halogen lamp. Chlorine gas molecules interacted with the zinc oxide, producing zinc chloride. This prompted a change in the relative permittivity and as a result, the residence wavelength of the surface plasmons.
A small amount of light travels within the cladding of the fiber. The resultant change in the transmission spectrum was measured using an Avantes AvaSpec-ULS3648-USB2 fiber-coupled spectrometer.
Figure 1 displays measured spectra as a function of chlorine concentration, demonstrating a detection range from 10 ppm to 100 ppm.
![Transmission spectra for various concentrations of chlorine gas (left) and the peak wavelength shift as a function of concentration (right). [1]](https://d12oja0ew7x0i8.cloudfront.net/images/Article_Images/ImageForArticle_19407_15935816855617314.png)
Figure 1. Transmission spectra for various concentrations of chlorine gas (left) and the peak wavelength shift as a function of concentration (right).1
Nano-Characterization by LSPR
LSPR spectroscopy is a formidable characterization tool, as it is extremely dependent on the nanostructure of particles or substrates. Researchers in Hungary utilized the fact that the line width of the LSPR absorbance spectrum is dependent on the uniformity of nanoparticles.2
This experiment involved sputtering four different thicknesses of gold nanoparticles with an estimated layer thickness of 7.5 nm, 12.5 nm, 15 nm, and 30 nm.
Utilizing an Avantes Avaspec-ULS2048-4DT-USB2 (four-channel high-resolution spectrometer) and an Avantes Avalight DHS halogen light source, the absorption spectra of the four samples, in the air (n=1), water (n=1.33), and oil (n=1.616) were measured.
The data obtained (shown in Figure 2) demonstrated a strong dependence on the relationship between the layer thickness and the FWHM of the surface plasmon resonance. This signifies a greater degree of variability in nanoparticle size as the layer thickness was increased.
![Fullwidth half maximum (FWHM) peak width of four sputtered gold nanoparticles of different thicknesses and a function of the environmental index of refraction. [2]](https://d12oja0ew7x0i8.cloudfront.net/images/Article_Images/ImageForArticle_19407_15935816963066009.png)
Figure 2. Full width half maximum (FWHM) peak width of four sputtered gold nanoparticles of different thicknesses and a function of the environmental index of refraction2.
Final Thoughts
SPR and LSPR spectroscopy can be used in an array of applications, including biological sensors, chemical sensors, and material characterization.
Additionally, high-resolution, low noise modular fiber-coupled spectrometers are an important tool in transitioning new SPR and LSPR sensors from the laboratory to the field and other environments.
When OEM systems, particularly those requiring high speed and continuous measurements such as biological and chemical hazard detection are required, Avantes’ AvaSpec instruments are an ideal option.
The spectrometers referred to above are also available as OEM modules. These can be incorporated into turnkey laboratory sensing devices, as well as operating as a supplement to existing laboratory equipment.
These units have a number of communication options: Ethernet, USB, and the native digital & analog input/output capabilities of the Avantes AS7010 EVO electronics board. This facilitates an enhanced interface with other devices.
The Avantes AvaSpec DLL software development package comes with sample programs in Delphi, C#, C++, Visual Basic, MatLab, LabView, and other programming environments. This allows users to develop customized code for their own applications.
References and Further Reading
- Usha, S.P., Mishra, S.K. and Gupta, B.D., 2015. Fabrication and characterization of a SPR based fiber optic sensor for the detection of chlorine gas using silver and zinc oxide. Materials, 8(5), pp.2204-2216.
- Bonyár, A., Wimmer, B. and Csarnovics, I., 2014, May. Development of a localised surface plasmon resonance sensor based on gold nanoparticles. In Proceedings of the 2014 37th International Spring Seminar on Electronics Technology (pp. 369-374). IEEE.
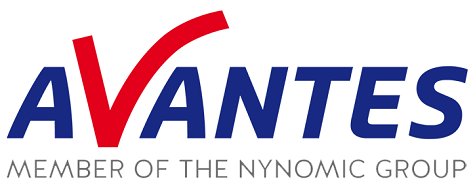
This information has been sourced, reviewed and adapted from materials provided by Avantes BV.
For more information on this source, please visit Avantes BV.