Key Issues
- Real-time, in situ, non-invasive analysis of catalysts on the molecular level
- Accurate measurement above 600oC
- Strong signal from active materials without interference from support
Introduction
Nitric oxide (NO) is a pollutant found in combustion emissions from both industrial sources and automobiles. Its presence can lead to smog formation in the lower atmosphere, while contributing to ozone depletion in the upper atmosphere. Despite considerable research, current approaches to the destruction of NO still possess major limitations.
In automobiles, the majority of the NO is eliminated alongside Carbon Monoxide (CO) and unburned hydrocarbons using ‘threeway catalysts’. One issue with these commercial catalysts, however, is that they are only able to function effectively over a narrow fuel/oxygen range. This has hindered the development of leaner burning engines which consume less fuel.
In most power plants, oxygen and a reducing agent like ammonia are commonly added to facilitate catalytic reduction of NO:
4NH3 + 2NO + 2O2 → 6H2O + 3N2 |
(1) |
However, an ideal reaction, especially for automotive use, would be direct catalytic dissociation of NO:
A catalyst for this dissociation reaction has not yet been developed which offers high enough activity at acceptable operating temperatures - a key issue being that catalytic activity is measured in moles per gram catalyst per second, usually scaling with increasing temperature.
The experiment outlined in this article utilizes Raman spectroscopy to investigate the use of barium oxide supported on magnesium oxide for NO dissociation. Under specific reaction conditions, extremely unusual behavior has been noted in this system.
For example, in cases where the barium loading is ≥11 mol %, the catalytic activity quickly rises with temperature, before sharply falling off.1 Additionally, this fall-off in temperature seems to depend on the partial pressures of NO and added O2.
Higher temperatures (>750 °C) result in catalytic reactivity beginning to recover, but the noticeable difference in slope (temperature dependence) suggests that different mechanisms are involved in these two temperature regimes (Figure 1).
Considerable research has been conducted with the goal of understanding this behavior, in terms of surface chemistry and surface phases.
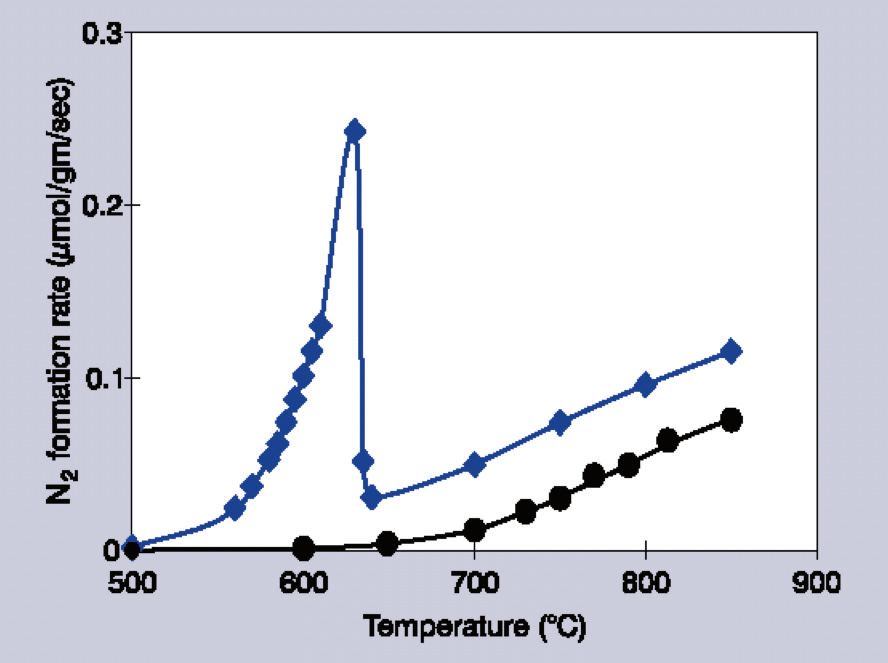
Figure 1. Decomposition rates of 1% NO/He over Ba/MgO catalysts. Circles indicate 1 mol % Ba/MgO, diamonds indicate 14 mol % Ba/MgO. Image Credit: Kaiser Optical Systems, Inc.
Analysis Methods
In theory, both Raman and FTIR could be employed to identify the different barium species that may be involved in this process. However, the most noteworthy chemistry appears at temperatures between 600 °C and 700 °C, and FTIR is not ideal for use above 500 °C due to excessive blackbody emission from the samples.
Experiment
Instead, in situ Raman measurements were obtained via a Kaiser Raman analyzer with 532 nm incident radiation. The analyzer’s high sensitivity is of particular importance, because surface concentrations of active species tend to be small, and as such low laser powers of just a few mW should be utilized to avoid excessive sample heating of a thermally sensitive reactive system such as this.
These samples do not fluoresce with visible excitation, so a short wavelength is preferred due to Raman’s signal intensity being proportional to 1/λ4. The use of short wavelengths also helps to prevent blackbody emission.
Both laser excitation and signal collection were completed using a Kaiser Raman probe, operating with a working distance of 50 mm. This setup’s high sensitivity enabled spectra to be acquired with only 2.5 mW of laser light on the sample. Further tests with thermocouples showed that this results in local temperature increases of <5 °C.
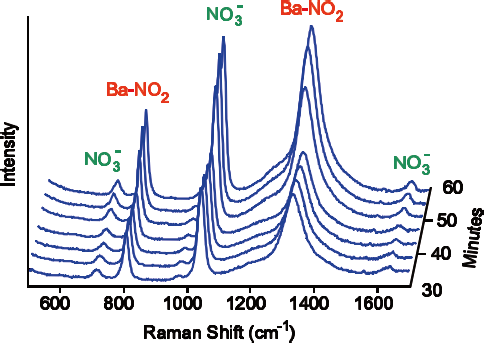
Figure 2. At 550 ºC a barium-nitro species builds up, corresponding to an increase in catalytic activity. Image Credit: Kaiser Optical Systems, Inc.
In each of the experiments described, 50 mg of the catalyst was supported on a fused-quartz frit through which the gases (O2, NO, He) flowed. This was enclosed within a low-volume (10 ml) cell to allow for fast re-equilibration after changes in gas mixture or operating temperature.
Results and Discussion
Depending on the specific operating conditions, Raman spectra could be seen for a range of different ‘BaNO’ species, including barium nitrite Ba(NO2)2, barium nitrate Ba(NO3)2 and a particular barium-nitro species - barium oxide with a nitro (NO2) ligand attached to the barium (Figure 2).
Several parameters such as operating temperature and gas partial pressures were varied around the activity peak, facilitating a move from the high-activity regime to the low-activity regime.
Spectral intensities for each of the ‘BaNO’ species was varied, with just the nitro species’ intensity closely correlating with the measured catalytic activity across the whole range of operating conditions. The results of this investigation indicate that the barium nitro species is actually the active intermediate responsible for this peak in catalytic activity.
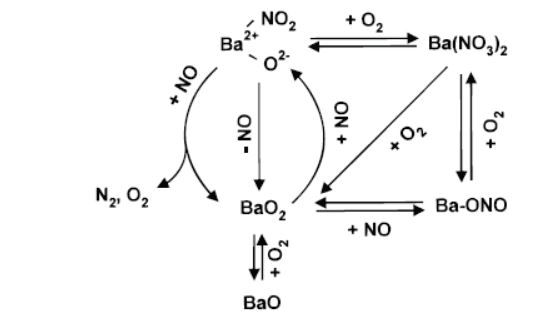
Figure 3. The catalytic reaction scheme. A barium-nitro reaction intermediate is formed by reaction of NO with barium peroxide (BaO2). Image Credit: Kaiser Optical Systems, Inc.
By actively monitoring the Raman bands due to the nitrate along with the bands assigned to crystalline barium peroxide, the noted complex dependency on O2 pressure could be understood, then incorporated into an overall reaction scheme.
Here, oxygen was found to play a dual role (Figure 3). Firstly, it creates barium peroxide, giving rise to the nitro species. Secondly, and conversely, excessive O2 was found to convert the active nitro species to the inactive nitrate.
The role of catalyst surface morphology is also being investigated. It seems that the active barium-nitro phase is not, in fact, a monolayer but instead behaves as though it is comprised of microcrystals. Raman spectroscopy is being employed to further study the chemistry of interaction between each of these phases.
Conclusion
As can be seen here, in situ Raman spectroscopy has been demonstrated as a vital tool in understanding a new catalytic mechanism for NO elimination. While this type of Ba/MgO catalyst alone does not seem to exhibit adequately high activity for eventual commercial use, this study has supplied valuable, detailed insight into the type and structure of catalytic intermediates that may cause NO decomposition.
References
- Xie, S.; Mestl, G.; Rosynek, M.P.; and Lunsford, J.H., “Decomposition of Nitric Oxide over Barium Oxide Supported on Magnesium Oxide. 1. Catalytic Results and In Situ Raman Spectroscopic Evidence for a Barium–Nitro Intermediate.” Journal of the American Chemical Society, Vol. 119, 1997, 10186.
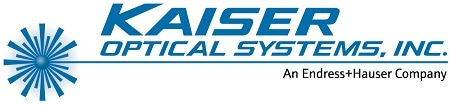
This information has been sourced, reviewed and adapted from materials provided by Kaiser Optical Systems, Inc..
For more information on this source, please visit Kaiser Optical Systems, Inc..