In this interview, AZoM talks to Alexander Maier Ph.D., a Senior Application Chemist at Nanalysis Corp, about the benefits of benchtop NMR in chemistry teaching, and runs through an example of using NMR to follow a typical organic reaction you might use in an undergraduate lab.
Nanalysis XPOD - Multistep Synthesis followed via Benchtop NMR
Nanalysis XPOD - Multistep Synthesis followed via Benchtop NMR from AZoNetwork on Vimeo.
What are the most important factors in helping students learn new methods, such as NMR?
“Knowledge cannot be passed verbally … students should be active participants in the learning process so they might construct knowledge within their own mind.” - this quote might be more relevant than ever. While we need to adapt to the pandemic situation and find suitable ways for teaching, we cannot ignore that meaningful learning is said to require three things: cognitive approaches (actual understanding of the material), affective approaches (the feelings associated with that material) and psychomotor learning (particularly important in a physical science like chemistry).
In laboratory courses, students can interact with colleagues and teachers directly. Instructions can be given on a more personalized level and in accordance with individual student’s needs. Additionally, guided inquiry and discovery sensations allow students to learn in the best possible way.
It is common practice to include characterization techniques within a laboratory course, but if we look at how often techniques are reported in the literature and compare this with how often students use them in a lab, there is a notable discrepancy.
The most-reported analytical method in publications is NMR spectroscopy.
Why is the use of NMR technology in teaching so limited?
High-field NMR spectrometers are not easily affordable or accessible due to high capital costs and operating expenditures, and because specially trained staff are required to maintain the liquid-cryogen-consuming instruments. The spectrometers at universities are often working to capacity, leaving little room for hands-on teaching and demonstrating. Also, accessibility may be limited if the NMR facility is not the same building as the lab course.
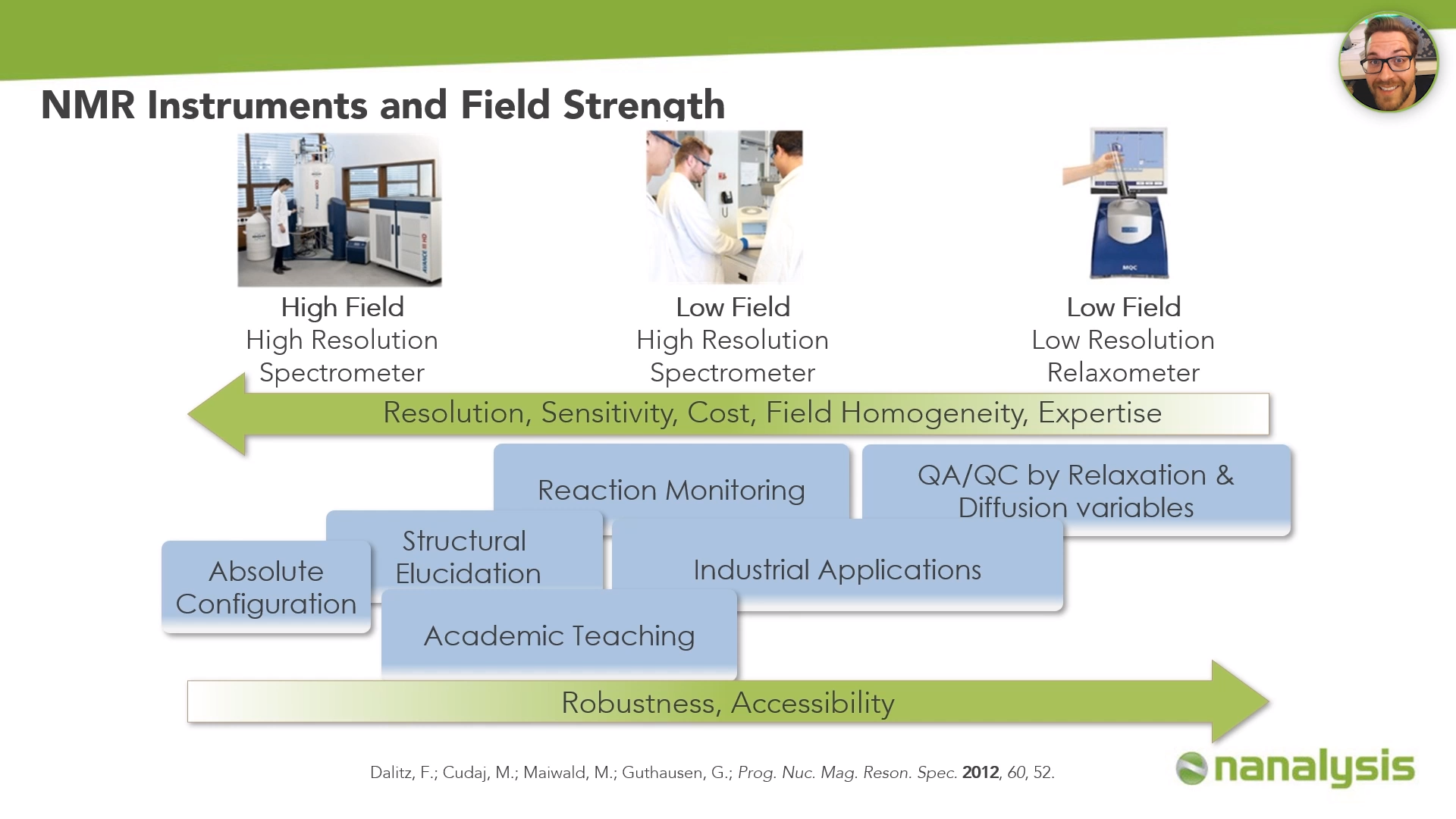
Because of this, NMR Spectroscopy is often introduced on a dry and theoretical level, where handouts are used for explaining the practical steps for NMR data acquisition instead of hands-on learning. The actual spectra come from either databases or previously recorded data from the TA; or, in a better scenario, students can pass their own samples to the analytical department and wait to receive their spectra back. Again though, students are missing out on the actual hands-on experience.
How has benchtop NMR helped give students access to hands-on learning with NMR?
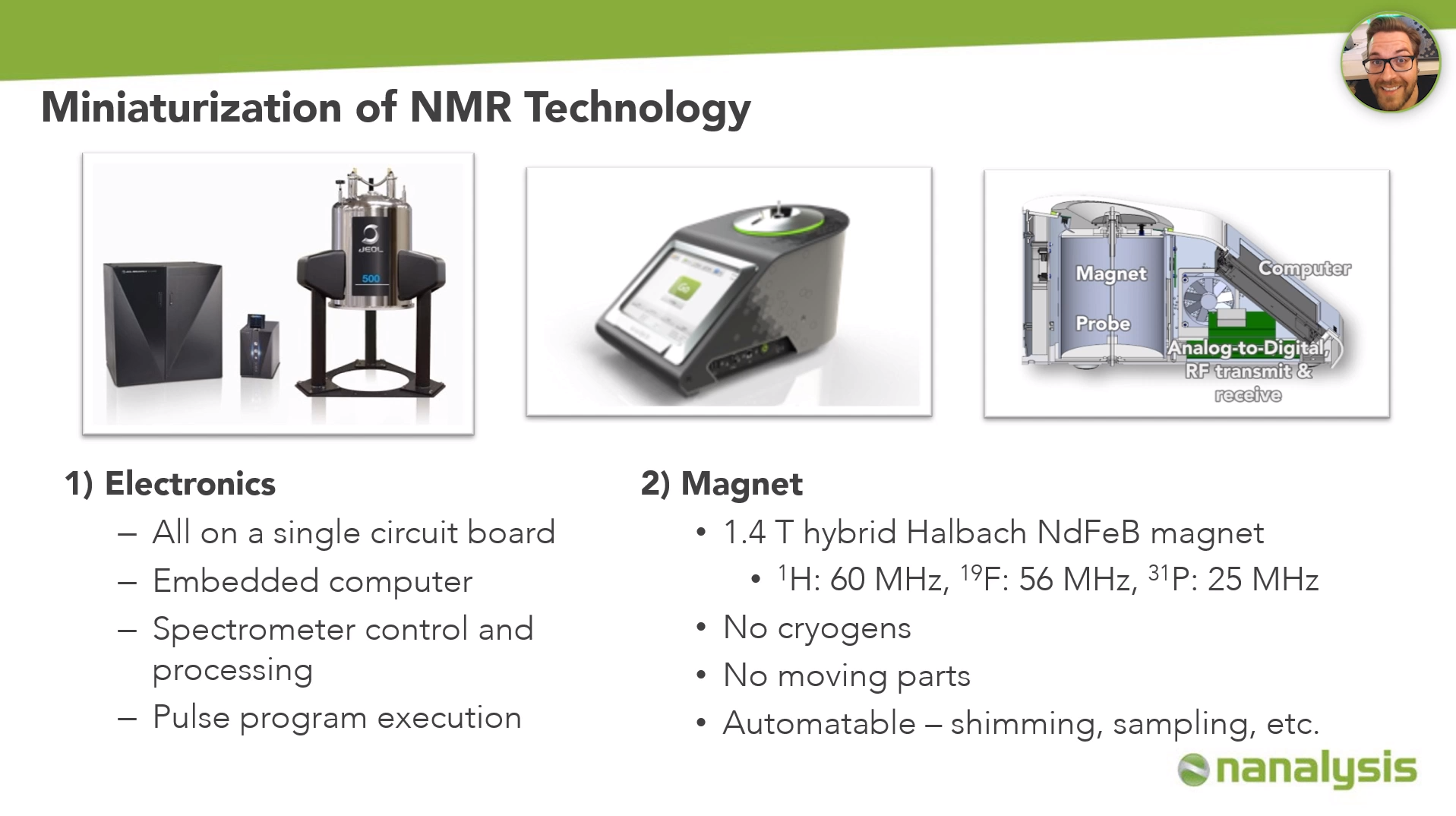
By equipping the teaching lab with a benchtop NMR, students can acquire their spectrum themselves, learning how to set up an experiment easily, and receiving the NMR spectrum directly. In this situation, the students can even learn how NMR specific parameters can be adjusted and what effect each of them has on the resulting spectrum.
Benchtop NMR devices have been available since the 2010s and result from the miniaturization of NMR technology. A regular high-field NMR spectrometer consists of three components: The cryogen cooled, superconducting magnet, the console - containing electronics allowing communication between the probe inside the magnet - and the computer, which makes the final component of this setup.
All of these components are combined in a single all-in-one enclosure. The electronics are mounted on one single circuit board. An internal computer is available, but you can also use an external computer for controlling the spectrometer and processing the NMR data.
As this system is based on permanent magnet technology, no cryogens are required and there are no moving parts, which could get stuck and cause interruption of measurement operations. Automatable features such as automated shimming allow the user to fully focus on the experiment.
How can benchtop NMR be classified and what can users expect from it?
Low-field high-resolution benchtop NMR spectrometers add a new layer of NMR technology, filling the gap between relaxometers and high-field NMR spectrometers.
While resolution and sensitivity increase with high-field instruments, so does cost and the level of expertise necessary to effectively work with these instruments. On the other hand, robustness and accessibility increase with relaxometers.
Our goal with benchtop NMR spectroscopy is not to replace high-field NMR, but rather to bring this powerful analytical technique to applications and settings where it has not been employed previously.
Benchtop NMR is affordable, accessible, and still features high resolution and sensitivity. This makes it an ideal instrument, not only for industrial applications or reaction monitoring but also for structural elucidation and academic teaching. Students are able to run their own samples next to the fume hood they have been working in on an easy to use a spectrometer, ultimately making NMR a rewarding technique to use.
What is the best way to introduce NMR spectroscopy to a student’s learning experience?
Understanding NMR Spectroscopy is a very important topic in chemical education, but there is little agreement on when or how to introduce this powerful characterization technique to undergraduates. Some educators introduce this as early as the first year, and some in the second year. One of the most common strategies is the introduction of NMR spectroscopy during the lecture component of introductory organic chemistry.
Some educators focus on the simplicity of symmetry and carbon NMR, whereas many start with proton NMR. With proton NMR, the key concepts require students to focus on three pieces of information simultaneously: (a) the chemical shift, representing the chemical environment of a nucleus; (b) the peak integration, which corresponds to the number of nuclei in the analyte signals; and (c) the multiplicity, reflecting the intramolecular connectivity of the compounds.
By introducing carbon NMR first, students can focus on understanding the chemical shift before dealing with integration and multiplicity in proton NMR. For example, the hydrochlorination of carvone can be used as a carbon NMR sample experiment. Here, only one of the four possible chlorinated products is formed. This can be unambiguously confirmed by counting the number of primary, secondary, tertiary, and quaternary carbon atoms as observed in the respective DEPT and 1D spectra.
Can you give our readers an example of a proton NMR sample experiment, typically demonstrated to students?
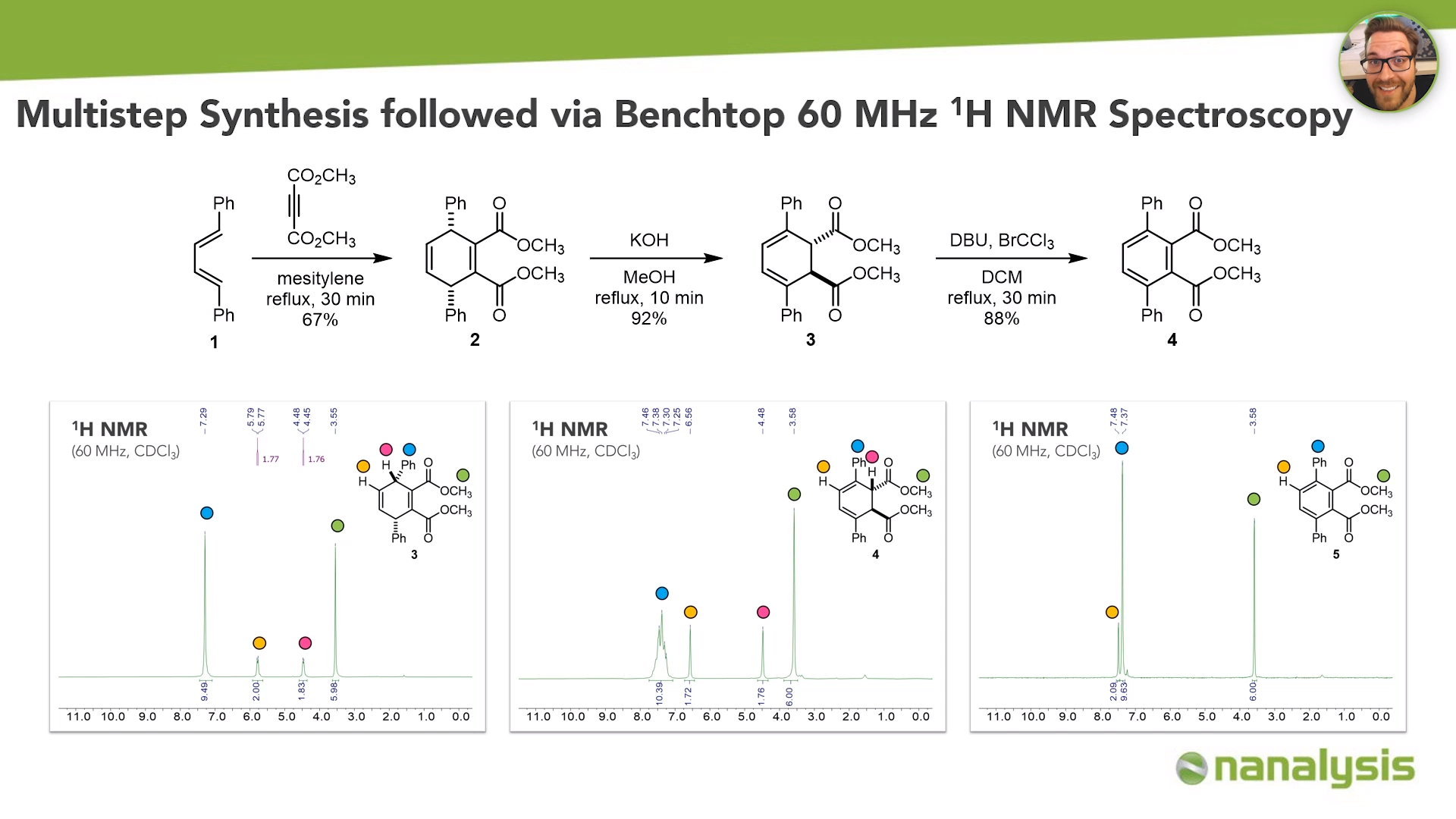
This procedure is based on an article from the Journal of Chemical Education and features a three-step synthesis of a para terphenyl derivative, including the renowned Diels-Alder reaction. Remarkably, these experiments can be performed within two to three quick lab sessions, including the acquisition of a 1D NMR spectrum for each step.
We start with the Diels-Alder reaction of diene and the acetylene dienophile in mesitylene at reflux for half an hour to generate dihydro terphenyl species in 67% yield. Isomerization of 1,4-dihdydro cyclohexadiene derivative under basic conditions in methanol leads to the 2,3-dihydro derivative in excellent yield. The dehydrogenation reaction employing DBU and bromotrichloromethane in DCM yields the para terphenyl in 88%, which corresponds to 54% yield over three steps.
Each of the products is obtained as a white solid and can successfully be characterized by benchtop NMR spectroscopy. Interestingly, the stereoselectivity of the Dies-Alder reaction can be rationalized by frontier molecular orbital theory and confirmed with the acquired spectra by the students.
In the 60 MHz NMR spectrum of the Diels-Alder product from the first step of the synthesis, the aromatic region of the signal of the phenyl groups was observed as a singlet at 7.29 ppm. The olefinic hydrogen atoms were observed at 5.77 ppm as a doublet with a constant of 1.8 Hz. The same coupling constant can be found for the doublet at 4.46 ppm, which identified the benzylic protons in vicinal position to the alkene ones. The methyl group signal at 3.55 ppm was clearly recognizable by the integration area of 6 and the multiplicity of a singlet due to the absence of neighboring protons.
It should be noted that not only can we assign all the hydrogen atoms for this first intermediate product in the synthesis, but also we can confirm that there is only one set of signals present, which means that the Diels-Alder reaction is stereospecific (as we all know from textbooks).
Due to the symmetry of the molecule, the number of theoretically possible different stereoisomers reduces from 4 to 2. The enantiomers can be aligned by rotation and therefore are identical. If there were two diasteromers present, we would see two signal sets in different intensities in the spectrum, as diastereomers can be differentiated via NMR spectroscopy. This observation perfectly agrees with the frontier molecular orbital theory.
The successful generation of isomerization products could also be confirmed by NMR. The aromatic hydrogen atoms appeared as a multiplet in the range of 7.46 to 7.25 ppm. Due to the conjugated double bonds, the alkene signal shifted downfield to 6.56 ppm in comparison to the previous spectrum. Both the alkene and the vinyl protons were then observed as singlets - not as doublets anymore. This was due to the absence of neighboring atoms. The methyl groups remain relatively unchanged at 3.58 ppm.
The final product - para terphenyl derivative 4 - showed a very clear NMR spectrum with two singlets in the aromatic region at 7.48 and 7.37 ppm, and a singlet in the alkyl region at 3.58 ppm, which can also be assigned to the methyl groups in the molecule.
Can you tell our readers more about the Diels-Alder Reaction and why this is important?
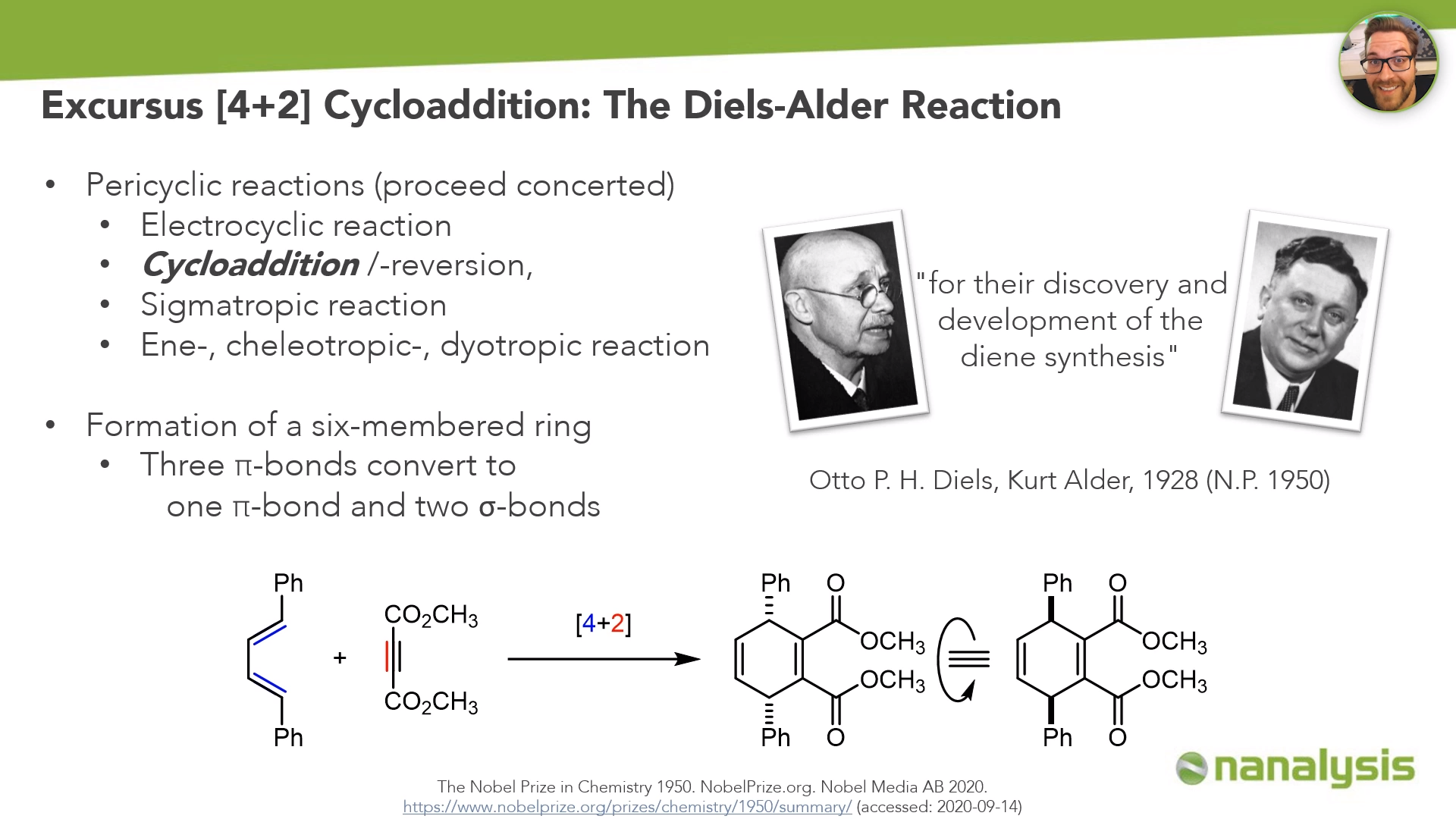
The Diels-Alder reaction is not only the key step in the synthesis discussed here, but it is also an excellent transformation worthy of study.
Otto Diels and Kurt Alder received the Nobel Prize in 1950 for their discovery and development of the diene synthesis. Today, almost every chemistry student knows the Diels Alder Reaction. It is formally a cycloaddition, which is a subcategory of the pericyclic reactions. Pericyclic reactions proceed concerted, and as well as the cycloadditions we can observe electrocyclizations, sigmatropic reactions, and others like the ene reaction.
In the Diels-Alder reaction three pi bonds – with four electrons coming from the diene and two electrons coming from the dienophile – convert to one new pi bond and two sigma bonds generating a six-membered ring.
In a diagram of this reaction, we would see the diene with the four different energy states ranging from zero to three nodes, alongside the two energy states of the dienophile. The diene counts four electrons, the dienophile counts two, so we can assign the HOMO and LUMO accordingly.
The presence of phenyl substituents on the diene and the electron-withdrawing methylester functions on the dieneophile would confirm a normal electron-demand Diels-Alder reaction. The HOMO of the electron-rich diene interacts with the LUMO of the electron-deficient dienophile because this energy gap is smaller than the one between the LUMO of the diene and the HOMO of the dienophile. With inverse-electron demand systems, this interaction is the other way around.
Because of the orbital coefficients of the diene and the dienophile, the interaction is suprafacial - this is allowed by the Woodward Hoffmann rules.
How can benchtop NMR be used to demonstrate a Diels-Alder reaction to students?
We recently performed a multistep synthesis, including the Diels-Alder reaction, and every intermediate product was analyzed and confirmed by 60 MHz benchtop NMR spectroscopy.
This sample experiment can also be utilized as a practical introduction of pericyclic reactions when working with students. Each step of the synthesis featured short reaction times and good yields, making it a suitable experiment even for short lab sessions in the organic curriculum.
The assignment of the NMR spectra was easy and in good agreement with the stereoselectivity of the Diels Alder reaction. Chemical shift, integration, and multiplicity can be discussed with students here, in regard to the molecular structures of the synthesized compounds.
Size, cost, and maintenance characteristics make benchtop NMR instruments an attractive option for providing first or second-year chemistry students with a hands-on introduction to NMR spectroscopy, with high respect to meaningful learning.
Are there any issues with low-field NMR instruments that educators should be aware of?
It should be noted that low-field instruments are more prone to produce NMR spectra with second-order effects. In contrast to first-order, spectra with second-order effects cannot easily be interpreted by the eye and some information is not extractable.
While there are plenty of compounds that do not show second-order effects at the low fields – such as the spectra I mentioned earlier - there are others that do. This can be concerning for educators who do not want to add this extra layer of complexity to any analysis.
To help address this concern, we recently submitted a paper designed to help educators overcome this limitation. In this paper, we presented a list of over 200 molecules from 20 compound classes whose NMR spectra can be visually inspected and interpreted using a 60 MHz instrument.
About Alexander Maier
Alexander Maier is a senior application chemist at Nanalysis’ European branch. He graduated with a Ph.D. in Organic Chemistry from Karlsruhe Institute of Technology, Germany, focusing on homogeneous catalysis primarily employing NMR spectroscopy analysis. Being part of the team for more than two years now, Alex wholeheartedly shares the corporate vision - making NMR spectroscopy accessible and bringing this powerful analytical method to places where it hasn’t been employed previously - and works on managing industrial collaborations and method validation for a wide range of applications.
This information has been sourced, reviewed and adapted from materials provided by Nanalysis Corp.
For more information on this source, please visit Nanalysis Corp.
Disclaimer: The views expressed here are those of the interviewee and do not necessarily represent the views of AZoM.com Limited (T/A) AZoNetwork, the owner and operator of this website. This disclaimer forms part of the Terms and Conditions of use of this website.