The use of granular materials and fine powders are commonplace in industrial applications.
These materials must be precisely characterized in order to properly control and optimize processing methods, with characterization methods typically relating to grain properties (for example, granulometry, chemical composition, or morphology) or to the behavior of the bulk powder (for example, flowability, blend stability, density, or electrostatic properties).
When it comes to analyzing the physical behavior of bulk powder, however, the majority of techniques commonly used in quality control or research and development laboratories are based on longstanding, often outdated measurement techniques.
GranuTools has spent the past decade updating these techniques to meet the requirements of contemporary production departments and research and development laboratories.
Measurement processes have become increasingly automatized, and the development of rigorous initialization methods has made obtaining interpretable and reproducible results easier than ever. Image analysis techniques have also been central in improving measurement precision.
A wide range of industries are already employing GranuTools’ instruments across a number of different fields; for example, food processing, additive manufacturing, pharmaceuticals and bulk material handling.
The use of powder-based additive manufacturing when working with metal powders is already common. This technique is garnering increasing interest for the manufacture of parts whose structure is often too complex to be achieved using standard machining.
A number of powder-based additive techniques have been developed; for example, the drop of binder, the localized fusion of particles in a powder bed, or the successive deposition of fused metal.
Regardless of the technique utilized, the powder must be conveyed through various process stages in the printer. As the powder flows through the printer, multiple contacts with the conveyor material and between the grains themselves will result in a charge build-up inside the powder. This is due to the triboelectric effect.
As charge density increases, an electrostatic related decrease of powder performance may arise, leading to issues with processability. A thorough understanding of the different process stages that contribute to charge build-up can enable ongoing improvements in the field of powder-based additive manufacturing.
In the study outlined here, GranuCharge has been used to investigate the electrostatic charging of a metal powder as it passes through the various parts of a Laser Metal Deposition (LMD) machine.
Powder samples were acquired at various locations of the powder conveying stage, facilitating an assessment of their individual influence on the charge build-up. This study also aimed to highlight the impact of powder distributor speed on any charge build-up.
GranuCharge
Electrostatic charges will be generated inside powders during a flow. This is a result of the triboelectric effect, which is essentially an exchange of charge at the contact between two solids.
As powder flows inside a device (for example, a silo, mixer, or conveyor), the triboelectric effect will occur at the contact between the grains themselves, as well as at the contact between the grains and the device.
Because of this, both the nature of the material used to construct the device and the characteristics of the powder used are important parameters.
The GranuCharge instrument (Figure 1) is able to automatically measure and precisely quantify electrostatic charges generated inside a powder during a flow and when in contact with a selected material.
This process involves the powder sample flowing inside a vibrating V-tube, falling in a Faraday cup that has been connected to an electrometer. The electrometer is used to measure any charge acquired by the powder as it flows inside the V-tube. A rotating or a vibrating device is used to feed the V-tube regularly, thus ensuring reproducible results.
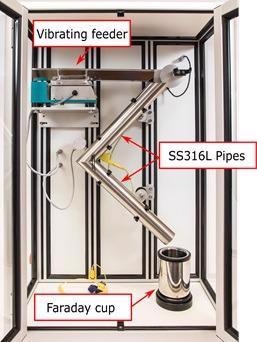
Figure 1. GranuCharge picture. Image Credit: Granutools
The LMD Machine
The study described here made use of a BEAM Modulo 400 LMD machine. The powder passed through different stages of conveying within the machine (Figure 2), initially flowing out of the powder distributor before being conveyed to the nozzle.
The powder then flows through the nozzle and is melted by the laser prior to deposition. Each of these stages has the potential for tribo-charging, leading to an increase in powder charge density.
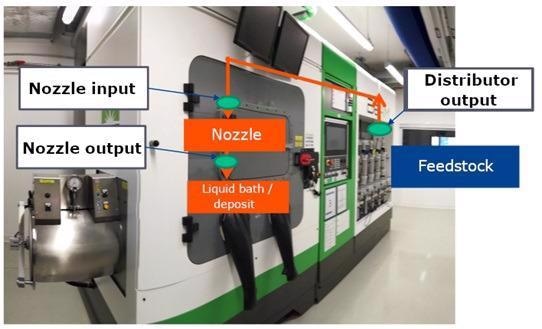
Figure 2. BEAM Modulo 400 used in this study. Image Credit: Safran
GranuCharge Analysis
Experimental Protocol
Powder charge density was measured using the GranuCharge at three process stages of interest: distributor output, before the nozzle and after the nozzle.
During each measurement, powder is poured into the GranuCharge cup directly via machine tubes (Figure 3). An extension was used in order to relocate the Faraday cup to outside of the GranuCharge.
The total charge and mass arriving inside the cup were accurately measured. Distributor speed was initially set to 0.68 rpm, 20% of the maximal speed of 3.4 rpm. Speed was varied in order to evaluate its impact on charge build-up inside the powder.
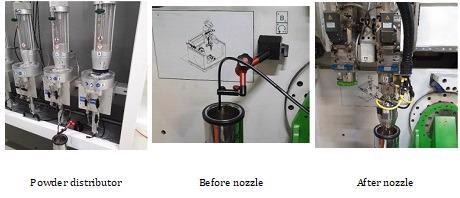
Figure 3. Printer locations at which the powder charge density is measured. Image Credit: Safran
Experimental Results
Figure 4 illustrates measured powder charge density at each stage of the process. At the distributor’s output, the powder exhibits a charge density of 0.39 nC/g. This shows that the flow through the distributor results in a charge build-up inside the powder.
The powder’s charge density just before the nozzle is significantly decreased, however. This would imply that charge dissipation takes place during conveying through the pipe between the distributor and the nozzle. Lastly, the flow through the nozzle continues to dissipate the charges, resulting in a slightly negative charge density at the output.
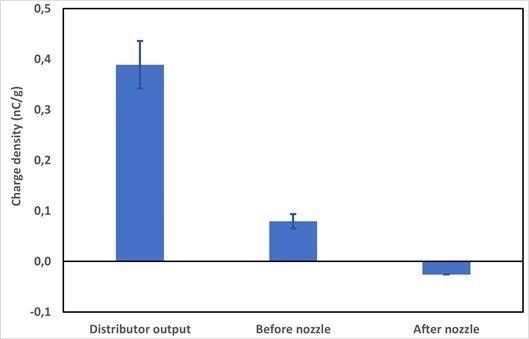
Figure 4. Measured charge density at the different process locations. Image Credit: Granutools
It was noted that conveying the powder through the pipes and the nozzle provides some benefit in terms of the tribo-charging because this process contributes to the dissipation of electrostatic charges. It was clearly identified that the distributor is the part of the process responsible for charge build-up.
Further investigation was undertaken on the impact of the distributor on the charging of the powder. Measurements were performed at a range of distributor speeds. Figure 5 displays charge density as measured with the GranuCharge.
Data is provided for several distributor speeds, which range from 10% to 30% of the maximal distributor speed (3.4 rpm).
These results suggest that the distributor speed has a considerable impact on charge build-up inside the powder - the higher the speed of the distributor, the higher the charge density of the powder at the distributor’s output. Figure 5 shows the best linear fit of this data.
A linear evolution of the charge density with the distributor speed was obtained for the range of investigated speeds. A powder processed at high distributor speed is therefore anticipated to exhibit an electrostatic related decrease in the powder’s performance.
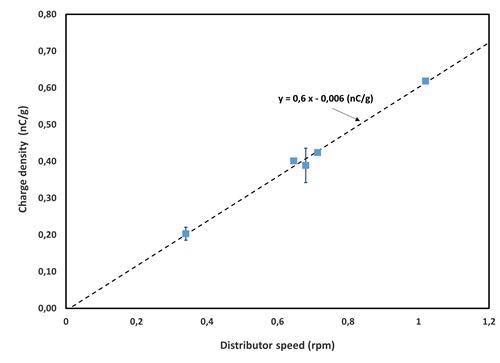
Figure 5: Influence of the distributor speed on the powder charge density. Dashed line represents the best linear fit of the data. Image Credit: Granutools
Conclusions
The GranuCharge instrument was used to investigate the impact of powder conveying in an LMD process on the charge build-up of an Inconel 718 powder. The powder’s charge density was measured at the output of the distributor, after the conveying between the distributor and the nozzle and after the flow through the nozzle.
The key findings of this study were that:
- The distributor prompts a charge build-up in the powder.
- Higher distributor speed results in a higher charge density in the output powder.
- Conveying to the nozzle and the nozzle itself contribute to a reduction in charge density.
The GranuCharge instrument facilitated the precise investigation of electrostatic charging of powders at various points throughout this process. This results in the potential for new optimization perspectives in terms of avoiding electrostatic charging throughout this process.
References
- Cascade of granular flows for characterizing segregation, G. Lumay, F. Boschin, R. Cloots, N. Vandewalle, Powder Technology 234, 32-36 (2013).
- Combined effect of moisture and electrostatic charges on powder flow, A. Rescaglio, J. Schockmel, N. Vandewalle and G. Lumay, EPJ Web of Conferences 140, 13009 (2017).
- Compaction dynamics of a magnetized powder, G. Lumay, S. Dorbolo and N. Vandewalle, Physical Review E 80, 041302 (2009).
- Compaction of anisotropic granular materials: Experiments and simulations, G. Lumay and N. Vandewalle, Physical Review E 70, 051314 (2004).
- Compaction Dynamics of Wet Granular Assemblies, J. E. Fiscina, G. Lumay, F. Ludewig and N. Vandewalle, Physical Review Letters 105, 048001 (2010).
- Effect of an electric field on an intermittent granular flow, E. Mersch, G. Lumay, F. Boschini, and N. Vandewalle, Physical Review E 81, 041309 (2010).
- Effect of relative air humidity on the flowability of lactose powders, G. Lumay, K. Traina, F. Boschini, V. Delaval, A. Rescaglio, R. Cloots and N. Vandewalle, Journal of Drug Delivery Science and Technology 35, 207-212 (2016).
- Experimental Study of Granular Compaction Dynamics at Different Scales: Grain Mobility, Hexagonal Domains, and Packing Fraction, G. Lumay and N. Vandewalle, Physical Review Letters 95, 028002 (2005).
- Flow abilities of powders and granular materials evidenced from dynamical tap density measurement, K. Traina, R. Cloots, S. Bontempi, G. Lumay, N. Vandewalle and F. Boschini, Powder Technology, 235, 842-852 (2013).
- Flow of magnetized grains in a rotating drum, G. Lumay and N. Vandewalle, Physical Review E 82, 040301(R) (2010).
- How tribo-electric charges modify powder flowability, A. Rescaglio, J. Schockmel, F. Francqui, N. Vandewalle, and G. Lumay, Annual Transactions of The Nordic Rheology Society 25, 17-21 (2016).
- Influence of cohesives forces on the macroscopic properties of granular assemblies, G. Lumay, J. Fiscina, F. Ludewig and N. Vandewalle, AIP Conference Proceedings 1542, 995 (2013).
- Linking compaction dynamics to the flow properties of powders, G. Lumay, N. Vandewalle, C. Bodson, L. Delattre and O. Gerasimov, Applied Physics Letters 89, 093505 (2006).
- Linking flowability and granulometry of lactose powders, F. Boschini, V. Delaval, K. Traina, N. Vandewalle, and G. Lumay, International Journal of Pharmaceutics 494, 312–320 (2015).
- Measuring the flowing properties of powders and grains, G. Lumay, F. Boschini, K. Traina, S. Bontempi, J.-C. Remy, R. Cloots, and N. Vandewalle, Powder Technology 224, 19-27 (2012).
- Motion of carbon nanotubes in a rotating drum: The dynamic angle of repose and a bed behavior diagram, S. L. Pirard, G. Lumay, N. Vandewalle, J-P. Pirard, Chemical Engineering Journal 146, 143-147 (2009).
- Mullite coatings on ceramic substrates: Stabilisation of Al2O3–SiO2 suspensions for spray drying of composite granules suitable for reactive plasma spraying, A. Schrijnemakers, S. André, G. Lumay, N. Vandewalle, F. Boschini, R. Cloots and B. Vertruyen, Journal of the European Ceramic Society 29, 2169–2175 (2009).
- Rheological behavior of β-Ti and NiTi powders produced by atomization for SLM production of open porous orthopedic implants, G. Yablokova, M. Speirs, J. Van Humbeeck, J.-P. Kruth, J. Schrooten, R. Cloots, F. Boschini, G. Lumay, J. Luyten, Powder Technology 283, 199–209 (2015).
- The flow rate of granular materials through an orifice, C. Mankoc, A. Janda, R. Arévalo, J. M. Pastor, I. Zuriguel, A. Garcimartín and D. Maza, Granular Matter 9, p407–414 (2007).
- The influence of grain shape, friction and cohesion on granular compaction dynamics, N. Vandewalle, G. Lumay, O. Gerasimov and F. Ludewig, The European Physical Journal E (2007).
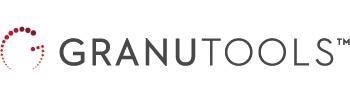
This information has been sourced, reviewed and adapted from materials provided by Granutools.
For more information on this source, please visit Granutools.