Interview conducted by Olivia FrostSep 15 2021
In this interview, Jonathan Lee, an Application Scientist at Gatan, talks to AZoM about improving sample Analysis using combined cathodoluminescence (CL) and energy dispersive spectroscopy (EDS).
Could you start by giving our readers a brief introduction to the instrumentation and experimental techniques that you commonly use at Gatan?
As an Application Scientist at Gatan, I'm asked to evaluate a very wide range of specimens, demonstrating what our tools can do for our customers. One day I might be analyzing future display technologies and, the next, meteorites from the earliest days of the solar system. It's important to me that customers are empowered to make the right choice for them when selecting which technique or instrument to use to analyze their samples. Working at Gatan, together with my colleagues at EDAX, I have the opportunity to use a complete range of analytical tools for electron microscopes, from imaging and electron energy loss spectrometers (EELS) for transmission electron microscopes to cathodoluminescence (CL) and electron backscattered diffraction (EBSD) systems for scanning electron microscopes (SEMs). However, one of the most common techniques I use is energy-dispersive X-ray spectroscopy (EDS or EDX).
In the SEM, an electron beam strikes a material and stimulates a range of electron signals are produced, including secondary electrons and backscattered electrons, that can be used to reveal sample morphology and topography. But there are a number of other signals that can be used, such as high-energy photons (X-rays), which can be used to provide compositional analysis via EDS, typically an EDAX Octane Elite EDS detector. This allows us to reveal the distribution of major, minor, and trace elements in a sample typically with a detection limit down to around 0.1 weight percent of the material under investigation.
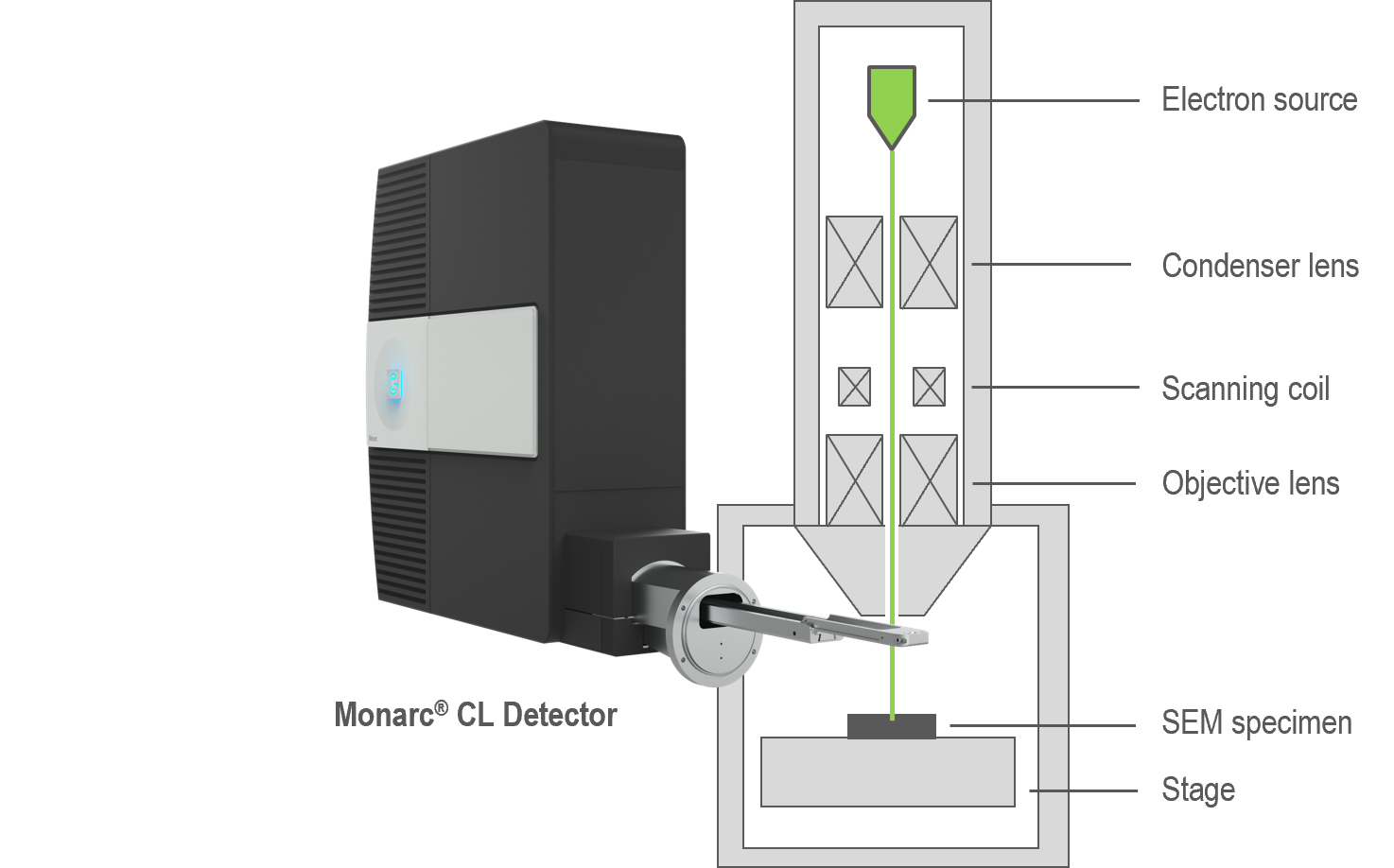
Schematic illustration of Monarc CL detector mounted to SEM chamber.
How does cathodoluminescence factor into your analyses using EDS?
Many processes are stimulated in a specimen when struck by an electron beam, but cathodoluminescence (CL) remains one of the most useful. CL is visible and ultraviolet light emitted from a specimen that can be used to reveal vital information about the electronic structure. This technique is commonly employed to characterize optoelectronic materials and devices, but in many rocks and minerals, we can use it to reveal trace element chemistry with a lower detection limit than in EDS - sometimes down to parts per million levels.
We can acquire this information by bolting a cathodoluminescence spectrometer to the microscope and extending a parabolic collection mirror above the sample to collect the light emitted from a specimen, coupling it into the detector. This allows us to correlate optical, chemical, and structural properties down to the nanoscale. When we couple CL and EDS together, these techniques provide a lot of complementary information.
When we place a paraboloid mirror above the sample, this would typically block out the line of sight needed by the EDS detector. We developed a collection optic to circumvent this issue, which allows a line of sight from the specimen to the EDS detector while maintaining significant light collection.
Could you give our readers an illustration of CL and EDS working in practice? How suitable is this combination of techniques for the analysis of meteorite samples, for example?
We recently used a combination of CL and EDS to investigate a CO3 carbonaceous chondritic (Miller range 090010) meteorite - a CO3 carbonaceous chondrite is carbonaceous (C), and Ornan (O) style with three (3) moderate to low shock pressures. Using the two techniques in parallel was a very powerful way of analyzing a precious sample.
The sample started life as a 2.5 kg stone collected in 2009 in the Antarctic during a routine search for meteorites. On the exterior of the grain, there was a black, generally glassy, fusion crust, and where this had been broken away in parts, we could see a dark, rusty brown interior with many fine-grain white inclusions. We were able to determine that the meteorite was composed of numerous chondrules, which ranged up to about 1.3 mm in size. There were also abundant, very fine-grained calcium aluminum-rich inclusions (CAIs).
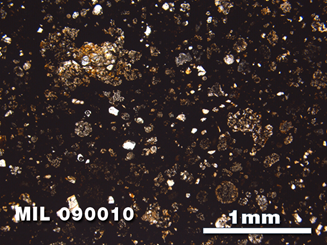
Plane-polarized light photomicrograph of the thin section of MIL 090010.
Image Credit: Meteorite Bulletin
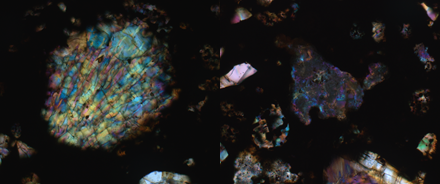
Optical microscopy images of sample section including (left) chondrule and (right) CAI.
As you can see, there was complex geological history to unravel as well as exposure to unusual conditions, so we needed to gain the maximum information in a systematic and streamlined way. Using a combination of EDS and CL measured simultaneously revealed mineral distributions and more subtle alteration and metamorphic processes.
Why is there so much current interest in CAIs and carbonaceous meteorites?
These are the most primitive meteorites that fall to Earth. As their name implies, they are rich in carbon and are therefore believed to have potentially been the building blocks for life on Earth.
There are also genetic links between CO and carbonaceous chondrites with primitive asteroids, such as Bennu and Ryugu - two Apollo family asteroids that currently have ongoing sample return missions.
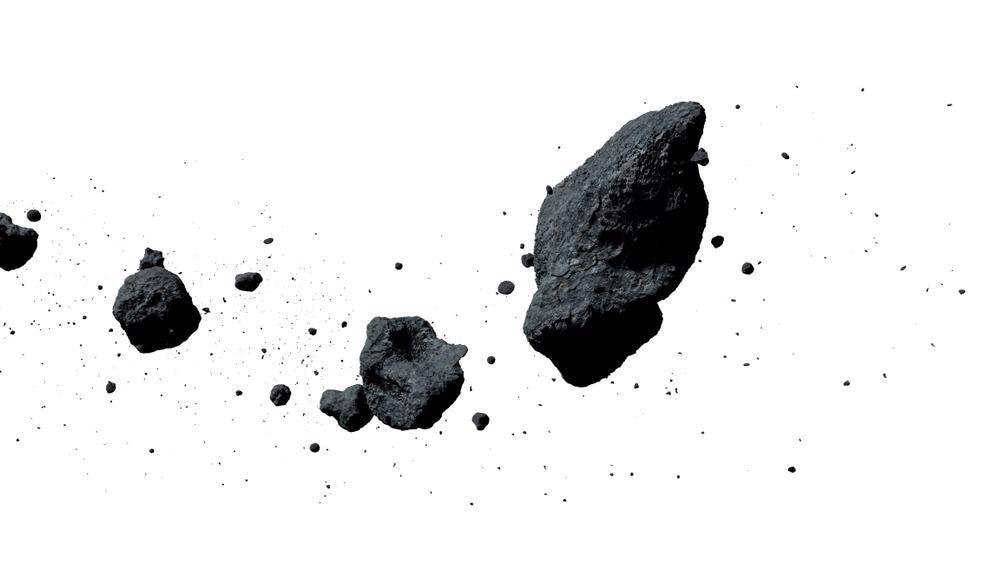
Image Credit: Shutterstock/Dotted Yeti
The JAXA-led Hayabusa 2 mission recently returned material from Ryugu, while the Osiris-REx mission led by NASA has just left Bennu and is expected to return in late 2023. Studying these carbonaceous chondrites will help guide future investigations of these return samples, helping us refine the techniques for future analyses.
Chondritic meteorites are composed of two components - chondrules and CAIs. Chondrules make up the dominant material, while calcium aluminum inclusions are less common.
Chondrules formed from distinctive molten droplets are believed to have formed either in the solar nebula or in impact processes on early asteroids. These are typically composed of magnesium-rich or forsterite-rich olivine.
Calcium aluminum inclusions formed even earlier than chondrules and are dated to 4.567 billion years ago, based on their lead isotopes.
These are composed of highly refractory mineral phases, including corundum, grossites, spinel, perovskite, hibonite, and melilite, which are thermodynamically calculated to have condensed from the nebular gas.
CAIs and chondrules represent the building blocks of asteroids, planetesimals, and ultimately planets. Understanding their complex formation history and any subsequent reworking is essential to resolving early planet formation history.
What benefits did the combination of CL and EDS bring to the characterization and analysis of CAIs?
Typically, CAI textures are characterized using backscattered electron imaging and EDS. Chemical maps (essentially their growth patterns) reflect their formation in the early solar system and any subsequent alteration and transportation through the stellar environment.
The CAI we investigated from MIL090010 CO3 carbonaceous chondrite was determined to be composed of spinel, hibonite, melilite, and calcium pyroxene with minor anorthite and perovskite. CL imaging revealed subtle zonation patterns that reflected this complicated crystallization and alternation sequence.
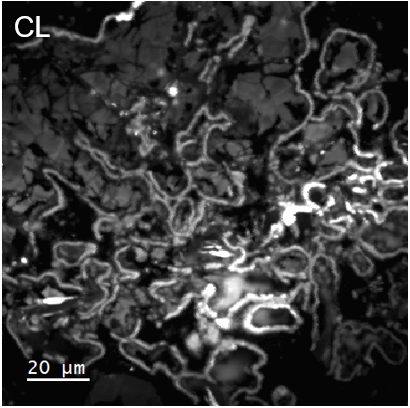
Unfiltered CL image of chondrule analyzed in this study.
Coupling EDS and CL gave us an in-depth view of the history of this grain - something which could not be resolved with just one technique.
When we looked at the EDS spectrum image to begin analyzing the data, it displayed a bandwidth around the oxygen line and revealed a great deal of compositional information.
To explore this in more detail, we mapped out the EDS data across the sample surface. For example, we mapped out the aluminum, calcium, and magnesium in the sample, stacking these into a composite image to look at where those elements were present and in what quantity.
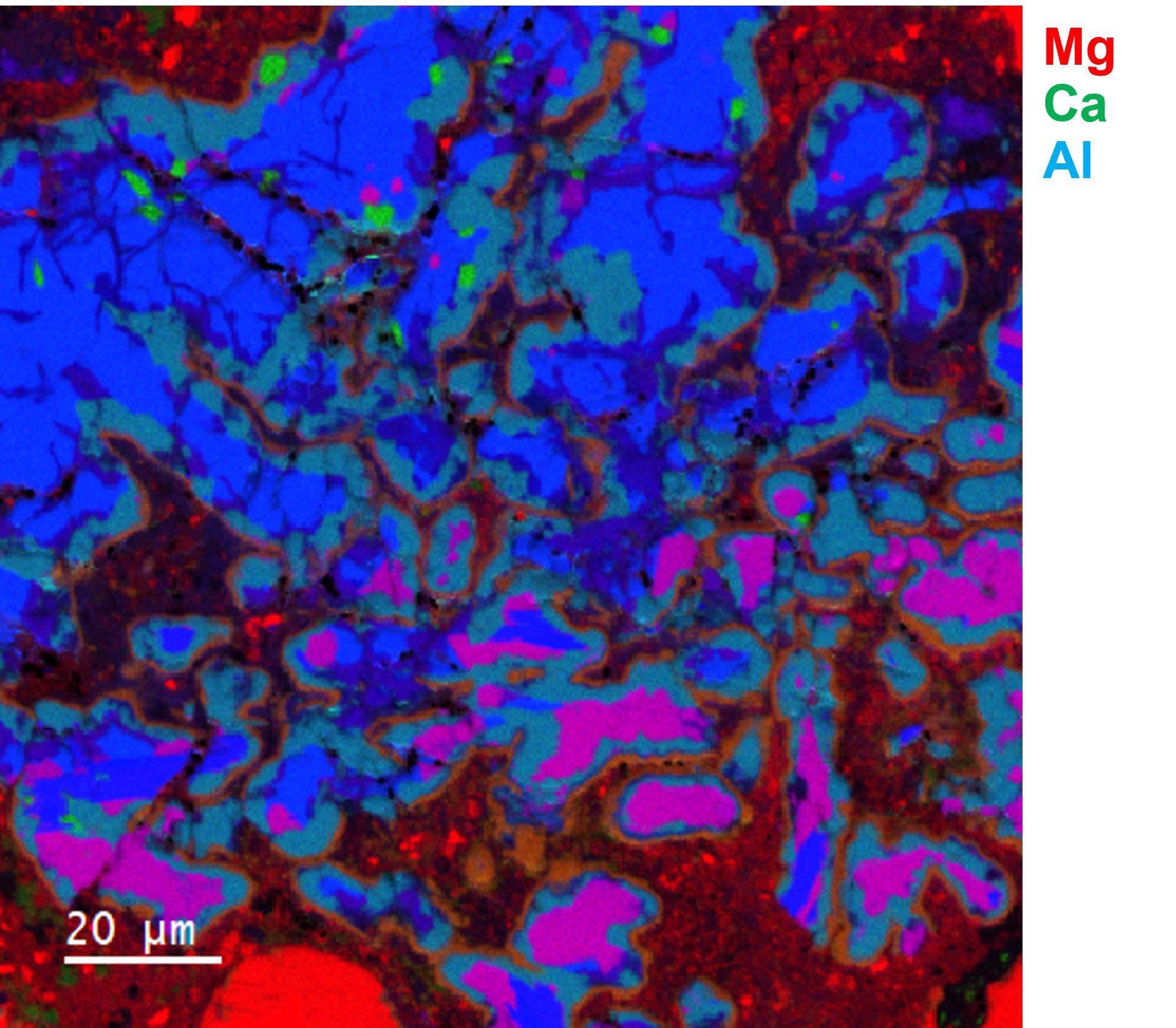
EDS map elemental quantification results displaying (red) magnesium, (green) calcium, and (blue) aluminum.
This approach revealed a number of calcium dominant, aluminum dominant regions, and magnesium dominant regions.
Unfortunately, EDS alone does not provide a lot of nuanced information. To address this, we then looked at the CL in a co-acquired spectrum image from the sample.
We immediately recognized that some of the spectra were unique to specific minerals; for example, spinel has a very characteristic CL emission spectrum related to its chromium attitudes in the crystal.
This was the most easily identified CL spectrum and gave us a starting point to identify unique spectra across the sample surface, and we used a multi-linear least-square fitting to locate and quantify those minerals across the sample surface.
Using this approach, we were able to identify and map calcic pyroxene, which tended to coat or shell over the other materials. Further investigation revealed two phases of melilite and hibonite grains, which tended to form the cores in the northern part of the specimen.
When using the element of quantification to verify the CL spectra employed in identifying the minerals in the specimen, we noticed that not all the melilite grains were equal.
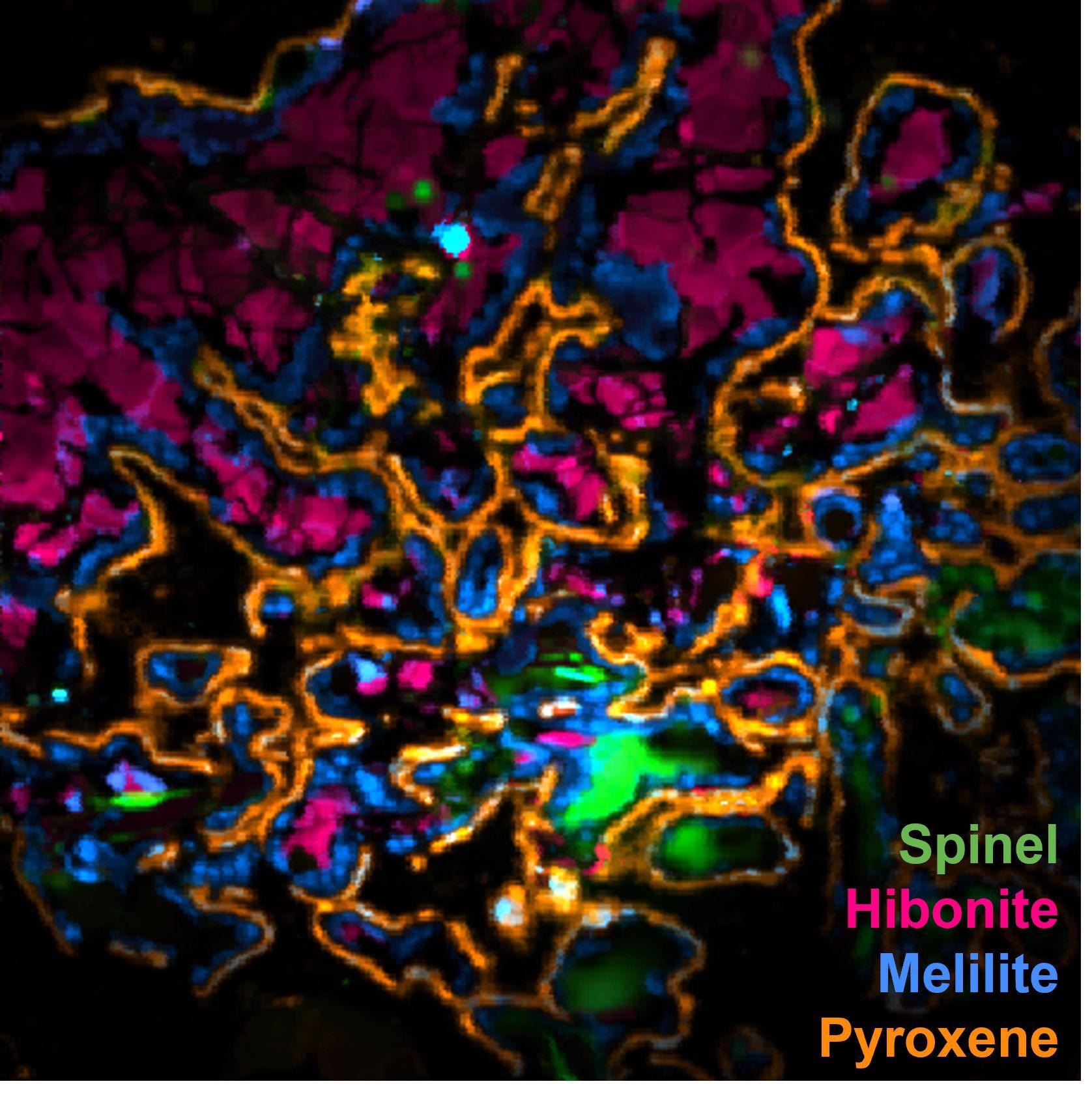
Mineral maps produced by CL spectrum image using reference spectra displaying (green) spinel, (magenta) hibonite, (blue) melilite, and (orange) calcic pyroxene.
Compositionally, two points representing melilite seemed nearly equivalent under EDS, but an investigation of the CL spectrum revealed a noticeable peak at 460 nm coming from one of the spots investigated.
A number of methods were used to try and map out the influence of that peak and better understand its role. We looked at where the peak was and investigated the difference with the background of the surrounding area to eliminate the influence of the background.
Doing so revealed that the peak was most prevalent towards the interior of the grains of the hibonite. We also discovered another peak at around 606 nm, which tended to be more prevalent in the melilite and pyroxene regions.
These elements seemed to exhibit a lot of influence that tended to vary across the sample surface. We were able to identify those materials to be Samarium inclusions in melilite and iron in the hibonite material.
How did you confirm that the CL image was successfully detecting these trace elements?
To ensure we were correctly detecting iron, we compared our results against an EDS iron map, then confirmed this again with our own measurements.
We concluded that the CL spectrum image was detecting trace elements because we know that CL can be affected by much lower concentrations of elements - on the order of parts per million.
We are actively building a library of CL spectra to assist in verifications such as this so that when researchers find something, they can use the library to identify those materials and what phase or condition they are in.
How useful are EDS and CL in investigating specific features like grain boundaries?
CL is an excellent technique for investigating grain boundaries – something that cannot be achieved in EDS. EDS offers high confidence in elemental quantification and element identification, but CL provides the opportunity to acquire more nuanced information, like grain boundaries.
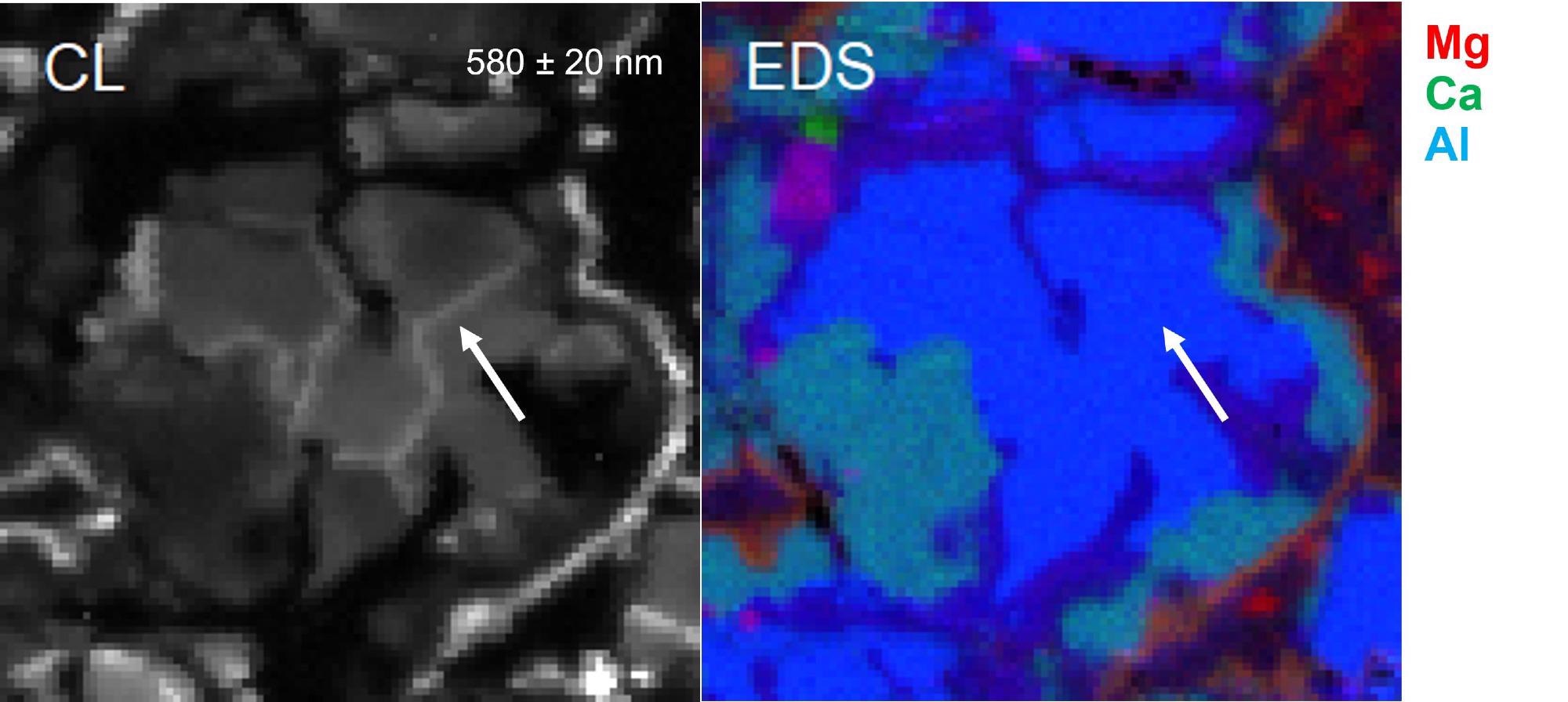
Comparison of CL and EDS demonstrating the grain boundaries detected by CL.
For example, the true color CL image revealed more subtlety in the melilite regions that were not visible in the EDS image.
We noted two domains in the CAI – one that is more spinel-rich and one that is hibonite-rich. The hibonite-rich domains appeared to have crystallized individual grains of hibonite, whereas the spinel-rich domain exhibited intergrowths of hibonite, spinel, and even some minor grossite.
This was particularly interesting as there seemed to be a control based on the primary crystallization on the microstructures that form.
We notice the development of sector zoning in the hibonite that was consistent with thermal alteration or metamorphism and subsequent alteration overprinting the hibonite grains.
This was all mantled by melilite, with the two melilite compositions appearing to be related to the primary grains, or the hibonite versus the spinel-rich intergrowths that are chemical compositions.
In the southern domain, we also saw minor anorthite that was crystallized, mantling the melilite. This was lacking from the northern domain, suggesting that primary chemistry controlled the phases and resulting alteration products. All of this material was found to be mantled by a thin layer of calcic pyroxene.
Combining these two techniques not only provided information about the crystallization or growth sequence and the alteration of those different phases, but it also allowed us to see subtle zonation patterns and grain boundaries, yielding insight that would not be evident using just one technique.
EDS accurately and quantifiably detects and identifies elemental species and constituents in a specimen. However, defects, grain boundaries, and trace elements are not observed using this technique. CL reveals the local charge recombination properties.
Grain boundaries slightly alter the recombination dynamics detectable by CL, so we can use this to identify different grain boundary and textural characteristics. The material can be identified through the emission spectrum because specific phases, such as spinel, have unique CL signatures that can be used to identify those phases.
Trace elements can also be determined at ultra-low concentrations - much lower than those detectable using EDS.
Combining EDS and CL spectral imaging offers the potential to reduce sample dosage, simplify the workflow and offer a perfect pixel correlation.
It is a very good technique for identifying complex zonation patterns in crystallization sequences in things like CAIs, which have a significant and protracted history in the early solar system.
What do you intend to use this data for, and how will this inform future research?
We will be using this data to guide subsequent or future analysis, for example, looking at nanoscale features of these growth domains via transmission electron microscopy. It can also be used to guide future dating analysis of stable isotopes, such as oxygen isotopes, or radiogenic isotopes, such as aluminum or magnesium.
This will also help deepen our understanding of the formation history of this grain, allowing us to link it to the region where it formed in the solar nebula and what thermodynamic events the different domains reflect.
About Jonathan Lee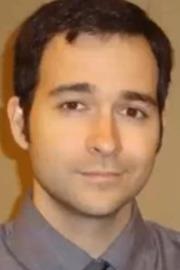
Jonathan Lee received his doctorate in physics from the University of Central Florida in 2018 studying the effects of high energy radiation on wide and narrow bandgap semiconductor materials using continuous and time-resolved cathodoluminescence (CL) techniques. More recently, he has been working with Gatan as an application scientist providing support for Gatan’s line of world class CL products. In this capacity he has demonstrated the capability of CL in several applications including defect mapping for failure analysis, energy-momentum mapping for photonics, and mineral mapping in geological (and exogeological) specimens.
About Gatan, Inc.
Gatan, Inc. is the world's leading manufacturer of instrumentation and software used to enhance and extend the operation and performance of electron microscopes. Gatan products, which are fully compatible with nearly all electron microscope models, cover the entire range of the research process—from specimen preparation and manipulation to imaging and analysis. The Gatan brand name is recognized and respected throughout the worldwide scientific community and has been synonymous with high-quality products and leading technology for more than 50 years. Gatan is headquartered in Pleasanton, California, U.S.A. For more information, visit: www.gatan.com.
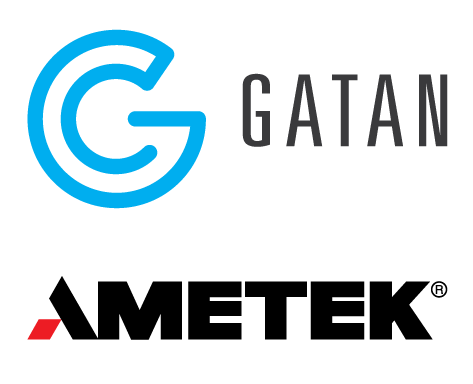
This information has been sourced, reviewed and adapted from materials provided by Gatan Inc.
For more information on this source, please visit Gatan Inc.
Disclaimer: The views expressed here are those of the interviewee and do not necessarily represent the views of AZoM.com Limited (T/A) AZoNetwork, the owner and operator of this website. This disclaimer forms part of the Terms and Conditions of use of this website.