In recent years, the field of spintronics has garnered much press and attention thanks to its untapped potential in applications like telecommunications, data storage and information processing.1
Switchable spin split electronic band dispersions with external stimuli are shown, among spintronics, in non-centrosymmetrics (NCS).
One such NCS is NaCu5S3 (space group P6322): a stable ferri-chiral compound that has been proven theoretically to exhibit chirality-dependent spin splitting within the electronic structure.
Experimental chirality determination using electron microscopy, on the other hand, has proven quite challenging. This difficulty arises as it is not possible to detect chirality (a three-dimensional structural feature) in a simple two-dimensional transmission electron microscope projection.
Materials and Methods
For uncompromised diffraction imaging at low-beam energies, the Stela™ hybrid-pixel camera is vital. Using the DECTRIS hybrid-pixel technology, Stela reveals difficult-to-detect diffraction details with the highest signal-to-noise ratio via electron counting.
Stela also leverages its high-dynamic-range sensor to capture low- and high-intensity diffraction peaks in a single frame.
Diffraction intensities observed from two enantiomers using convergent beam electron diffraction (CBED) will differ based on the violation of Friedel’s law in chiral crystals.2 Chiral identification, therefore, is possible using the intensity asymmetry in Bijvoet pairs of reflections on the correctly-chosen zone axis.
Intensity differences such as these can be very subtle, making them difficult to detect. This complexity thus requires an electron detector that boasts both a high signal-to-noise ratio and dynamic range. CBED patterns were collected from synthesized NaCu5S3 crystals in a JEOL Grand ARM operated at 80 kV using the Stela camera. A beam stop was not required.
The camera’s high-dynamic-range (HDR) mode collected the 512 x 512 pixels CBED pattern, with a total exposure time of 0.5 seconds (pixel size of 0.092 nm-1). A single frame was therefore used to collect both central beam (without saturation) and FOLZ discs.
![Experimental CBED patterns of (a) FEC-1 and (d) FEC-2 NaCu5S3 along [3301] zone axis, showing the asymmetric intensity distribution of FOLZ reflections in two enantiomers. (b) and (c) high-magnified and contrast-enhanced images of critical discs in the red-marked box in (a) and (d): the intensity profile of critical discs shows the asymmetric brightness. (e) intensity profile along the dashed yellow line in (d): no saturation (32 bit) at the central beam location (log scale).](https://d12oja0ew7x0i8.cloudfront.net/images/Article_Images/ImageForArticle_21191_16430925635383562.jpg)
Figure 1. Experimental CBED patterns of (a) FEC-1 and (d) FEC-2 NaCu5S3 along [3301] zone axis, showing the asymmetric intensity distribution of FOLZ reflections in two enantiomers. (b) and (c) high-magnified and contrast-enhanced images of critical discs in the red-marked box in (a) and (d): the intensity profile of critical discs shows the asymmetric brightness. (e) intensity profile along the dashed yellow line in (d): no saturation (32 bit) at the central beam location (log scale). Image Credit: Gatan Inc.
Summary
Leveraging the Stela hybrid-pixel camera’s high-quality diffraction imaging capability facilitated a study of chirality in spintronics. The Stela was used to capture deeply subtle difference/intensity asymmetry between Bijvoet pairs in CBED patterns.
Gatan, Inc. is the global market-leading manufacturer of software and instrumentation used to improve and extend electron microscopes— ranging from imaging and analysis to specimen preparation and manipulation.
Credit(s)
Gratitude is owed to Northwestern University Chi Zhang, Ken Poeppelmeier, Vinayak P. Dravid, and Roberto dos Reis. The EPIC facility of Northwestern University’s NUANCE Center was used in this work, which has received support from the SHyNE Resource (NSF ECCS-2025633), the IIN and Northwestern’s MRSEC program (NSF DMR-1720139).
References
- W. W. Chow and S. Reitzenstein, Quantum-Optical Influences in Optoelectronics—An Introduction, Applied Physics Reviews 5, 041302 (2018).
- J. M. Bijvoet, A. F. Peerdeman, and A. J. van BOMMEL, Determination of the Absolute Configuration of Optically Active Compounds by Means of X-Rays, Nature 168, 271 (1951).
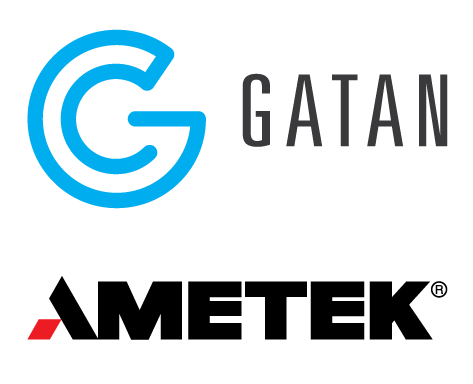
This information has been sourced, reviewed and adapted from materials provided by Gatan Inc.
For more information on this source, please visit Gatan Inc.