Sponsored by AvantesReviewed by Emily MageeMar 7 2023
Nonthermal and thermal plasmas are used in the industrial, engineering, medical, and research industries. Plasma composition is an important parameter in any plasma process. Using optical emission spectroscopy can provide accurate, and near-real-time monitoring of plasma in chambers and plasma-contacting substrates, thereby facilitating inline process monitoring and optimization.
A gas is called a “plasma” if a significant portion of its molecules has undergone ionization, creating a variety of excited atoms, ions, and molecules.
High-energy electrons released from atoms and ions can freely flow throughout the plasma. When colliding, these energetic electrons can impart some of their energy to neutral atoms and molecules.
Neutral atoms and molecules release photons of light when they relax back to their ground state. The energy of the photons, which in turn depends on the energy difference between the excited state and ground state of the neutral atoms and molecules, determines the color of the light emitted.
As unbound charged particles are electrically conductive and can be shaped and contained by magnetic fields, their presence alters the bulk electromagnetic characteristics of plasmas.
Moreover, ionization makes plasmas highly reactive, but thermal plasmas’ high temperatures (and high particle velocities) are sufficient to vaporize substrates.
A variety of pressures and temperatures can lead to the development of plasmas. Plasma behavior can be significantly changed, and plasmas suitable for various purposes can be created by adjusting these parameters and altering the chemical species present in plasma.
These factors must be precisely controlled for processes to remain stable, dependable, and reproducible.
Key Plasma Parameters
Plasmas can be generally classified in terms of pressure, temperature, and the chemical species in the plasma in industry and research.
Plasma temperature can be expressed in kelvin, much like that of gases and other substances, and it is directly related to the thermal kinetic energy per particle. However, the effective temperature of the ions in plasma can occasionally be considerably different from that of the electrons due to the difference in mass between ions and electrons.
Electron temperature rises in electromagnetically confined plasmas because energy is often transmitted to electrons considerably more effectively than to ions. The rate of energy transfer between electrons and ions, and between the ions and the environment, controls the temperature of the ions.
Ions quickly transmit heat to their surroundings in nonthermal (or “cold”) plasmas before reaching high temperatures. The plasma has a generally low temperature due to the bulk of thermal energy being stored in electrons rather than ions.
Nonthermal plasmas can be employed in medical applications in combination with heat-sensitive materials and are frequently touch-safe.1
Thermal plasmas, on the other hand, are when the ion temperature approaches the electron temperature. Thermal plasmas are widely used in etching, polishing, and surface-texturing operations because their particle energies are often high enough to ablate surfaces. Other uses for thermal plasmas include accurate coating deposition in physical and chemical vapor deposition procedures.2,3
Since decreasing pressure slows down electron-ion recombination and reduces collisions with other molecules, many plasma applications are performed in vacuum environments. This increases the mean free path of plasma particles. However, recent advancements in plasma technology have made it possible for more plasma applications at atmospheric pressure.
The process gas used has a significant impact on the chemical species present in the plasma. However, the composition is also significantly influenced by pressure and temperature. A change in the chemical species will also impact the color since the plasma’s color is directly tied to its composition.
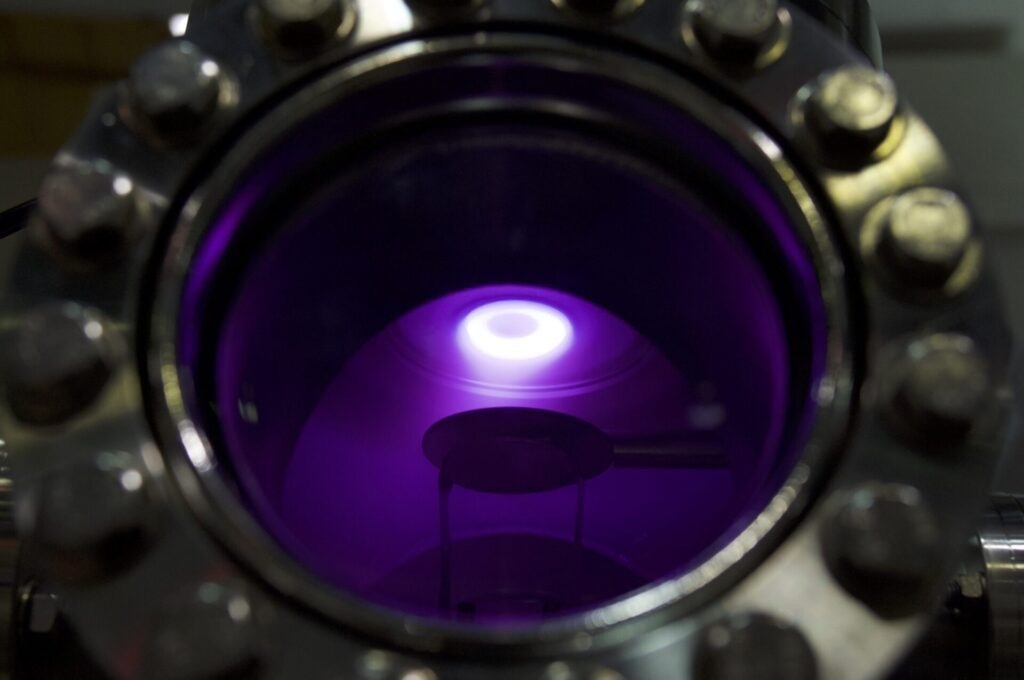
Plasma Vacuum Chamber. Image Credit: Avantes BV
Optical Spectroscopy for Plasma Analysis
In all types of plasma applications, plasma composition is an essential element. Characterizing molecular species in the plasma and on surfaces ensures precise process control and reproducibility in industry, engineering, and medicine.
“Optical emission spectroscopy” refers to a broad range of methods for measuring optical absorption/emission spectra or the light emitted when a sample is excited. An essential process for analyzing plasma is optical emission spectroscopy, which provides a precise non-contact characterization of the species present based on the color of the plasma.
Furthermore, optical spectroscopy is suitable for applications susceptible to contamination and in extreme thermal plasma environments. A wide range of plasma applications can benefit from the near-real-time feedback that optical spectroscopy offers, which enables inline process monitoring and quick, responsive process optimization.
Vacuum Plasma Applications
The plasma etch process in semiconductor manufacturing is one of the plasma’s most prominent vacuum applications.4 This sophisticated application carefully regulates removing material from a surface to create patterns on silicon wafers.
Plasma etching in semiconductor devices calls for exceedingly accurate endpoint detection, i.e., the monitoring of the reaction’s progress, and it is also highly prone to contamination.
Due to these factors, plasma etching necessitates exceedingly precise plasma analysis. The speed, stability, and resolution required for inline process control in plasma etch applications are provided by optical emission spectroscopy, generally spanning the near-infrared to near-ultraviolet regions of the spectrum.
Several plasma-driven chemical vapor deposition (CVD) and physical vapor deposition (PVD) methods, including sputtering, plasma-enhanced chemical vapor deposition (PECVD), and ion beam deposition, also employ vacuum plasma systems for the deposition of coatings and thin films.
Due to their use of highly reactive plasma species and high plasma energy, these procedures frequently require severe operating conditions. As optical emission spectroscopy is a non-contact method, it is essential for plasma analysis in these applications. Accurate standoff measurements of the process environment can be made using optical fibers.
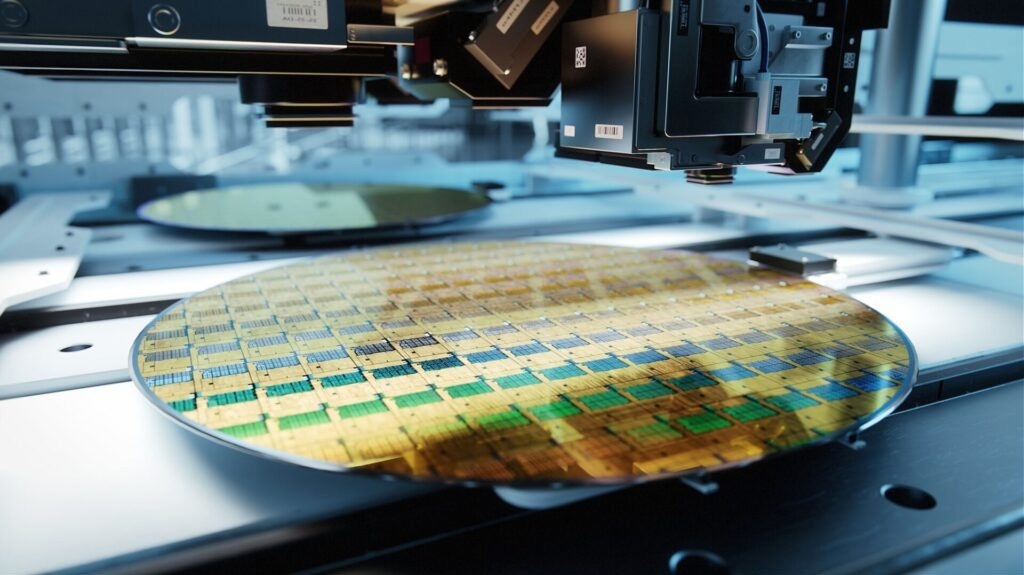
Silicon wafer inline process control. Image Credit: Avantes BV
Atmospheric Pressure Plasma Applications
Nonthermal atmospheric pressure plasmas have been used in many medical procedures since they efficiently sterilize instruments and tissues. Moreover, they can be adjusted for multiple functions such as cell detachment, wound healing, and genetic transfection.1,5,6
In medical plasma applications, where disposable fiber optic interfaces provide affordable disposable testing compatible with hygienic in vivo applications,7 fiber optic spectrometers are especially useful.
Atmospheric plasmas are frequently utilized for surface polishing and modification. While reactive atom plasma (RAP) has been demonstrated to help figure out optical components, electrolytic plasma polishing (EPP) includes the generation of air-pressure plasmas in electrolytic baths for finishing metal surfaces.8,9
Optical emission spectroscopy offers a quick and affordable plasma analysis option for inline analysis and process control in various applications.
In a variety of additional atmospheric pressure plasma applications, optical emission spectroscopy is essential for plasma analysis due to near real-time feedback:
- A standard method for elemental analysis is inductively coupled plasma mass spectrometry (ICP-OES), in which a sample is ionized by atmospheric pressure plasma before being subjected to spectroscopic analysis
- A fast-expanding field called plasma aerodynamics aims to employ plasmas to regulate aerodynamic processes. Managing laminar to turbulent transitions, lowering drag, and preventing local heating are a few examples.10,11
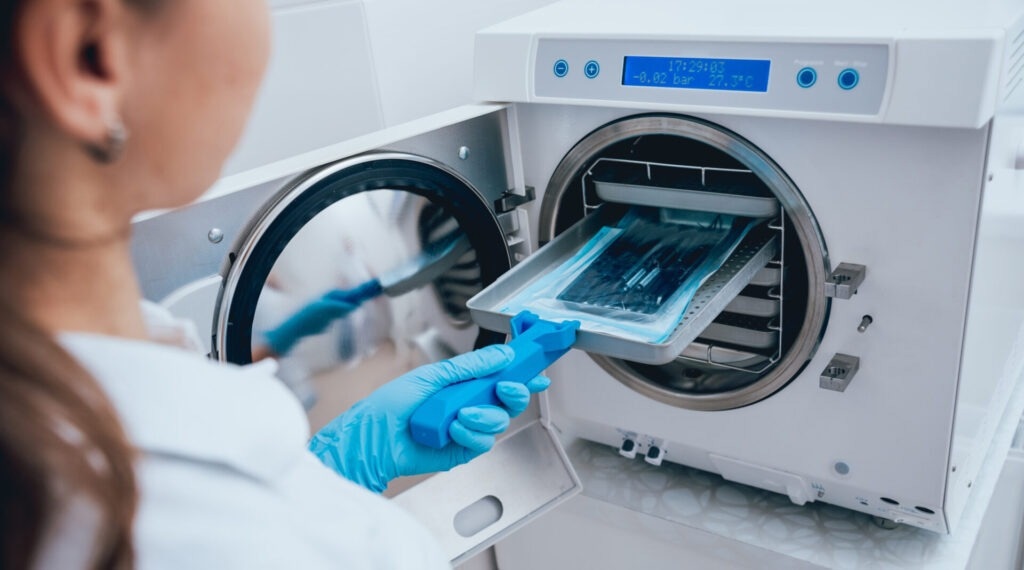
Sterilizing medical tools. Image Credit: Avantes BV
Avantes Optical Spectroscopy Systems for Plasma Analysis
While working in hostile plasma conditions and avoiding contamination, optical emission spectroscopy is the ideal non-invasive measurement technique.
However, a high number of closely spaced emission peaks often characterize plasmas. An optical spectroscopic device with a high spectral resolution is necessary to separate these peaks.
For advanced plasma applications, multichannel spectrometer systems from Avantes offer an extraordinarily high spectral resolution, even making it possible to characterize electrically excited molecules' vibrational and rotational distribution functions.
While CCD sensors have long dominated spectroscopy applications, more recent devices, such as the Avantes AvaSpec-ULS4096CL-EVO, use CMOS sensors to perform more quickly and accurately.
The AvaSpec-ULS4096CL-EVO is ideal for even the most demanding plasma analysis applications since it offers integration times as low as 9 μs and up to 0.05 nm resolution in the 200 nm to 400 nm region utilizing a 3600-groove density grating.
References and Further Reading
- Fridman, G. et al. (2008) Applied Plasma Medicine. Plasma Processes and Polymers, 5, pp. 503–533
- Martinu, L. & Poitras, D. (2000) Plasma deposition of optical films and coatings: A review. Journal of Vacuum Science & Technology A: Vacuum, Surfaces, and Films, 18, pp. 2619–2645
- Eskildsen, S. S., Mathiasen, C. & Foss, M. (1999) Plasma CVD: process capabilities and economic aspects. Surface and Coatings Technology, 116–119, pp. 18–24
- Darnon, M. (2017) Plasma Etching in Microelectronics. in Plasma Etching Processes for CMOS Devices Realization 23–58 (Elsevier, 2017). doi:10.1016/B978-1-78548-096-6.50002-X
- Bekeschus, S., von Woedtke, T., Emmert, S. & Schmidt, A. (2021) Medical gas plasma-stimulated wound healing: Evidence and mechanisms. Redox Biol, 46, p. 102116
- Scholtz, V., Pazlarova, J., Souskova, H., Khun, J. & Julak, J. (2015) Nonthermal plasma — A tool for decontamination and disinfection. Biotechnology Advances, 33, pp. 1108–1119
- Medical/Biomedical. Avantes Available at: https://www.avantes.com/applications/markets/biomedical-medical/
- Huang, Y. et al. (2021) Principle, process, and application of metal plasma electrolytic polishing: a review. Int J Adv Manuf Technol, 114, pp. 1893–1912
- Jourdain, R., Castelli, M., Morantz, P. & Shore, P. (2012) Plasma surface figuring of large optical components. 8430, p. 843011
- Jonathan, P., Thomas, M. & Sergey, L. (2015) Plasma Aerodynamics: Current Status and Future Directions. AerospaceLab Journal Issue 10, p. 6.
- Mohamed, A.-A. H., Fadhlalmawla, S. A. & Almarashi, J. Q. M. The shift in the laminar-to-turbulent transition flow mode in atmospheric pressure plasma jet. Plasma Processes and Polymers n/a, p. e2200145
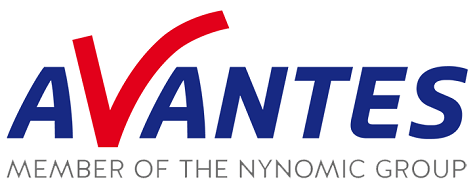
This information has been sourced, reviewed and adapted from materials provided by Avantes BV.
For more information on this source, please visit Avantes BV.