Proton transfer reaction – mass spectrometry, or PTR-MS, is a technique that is extensively used for monitoring low-concentration volatile organic compounds (VOCs) in real time.
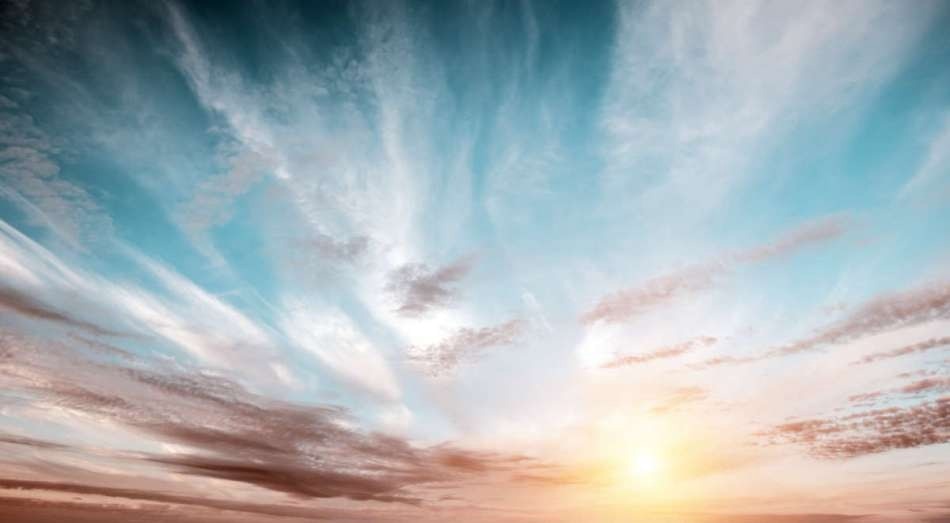
Key Advantages of Proton Transfer Reaction Mass Spectrometry (PTR-MS)
- Hundreds of VOCs can be detected simultaneously and in real time
- Sample preparation is not required: The gaseous sample is directly examined
- Lowest limits of detection (LODs) available for analyzing VOCs in real time
- VOC concentrations can be quantified, even without calibration with standards
- VOCs can be detected in solid or liquid samples through headspace analysis or other interfaces
- Fragmentation and spectral complexity are reduced by soft chemical ionization
The Principle of Proton Transfer Reaction Mass Spectrometry (PTR-MS)
The gaseous sample comprising of the VOCs to be examined (for instance, ambient air) is directly sampled into the PTR mass spectrometer without preparing any samples.
The PTR ionization source is the first stage of the PTR mass spectrometer. The gas-phase VOC molecules in this ionization source are ionized by transferring a proton from H3O+ to the VOC molecules:
VOC + H3O+ → VOC·H+ + H2O
The above reaction produces the VOC ions (VOC·H+) that are subsequently detected with a mass analyzer. The concentrations of the VOCs in the sample can be identified and determined using the PTR-MS data.
Which Compounds Can Be Detected and Monitored with PTR-MS?
The proton transfer reaction can take place only when the VOC molecule exhibits a higher proton affinity than water. This condition is satisfied by many VOCs, and therefore they can be detected by PTR-MS, as shown in Figure 1.
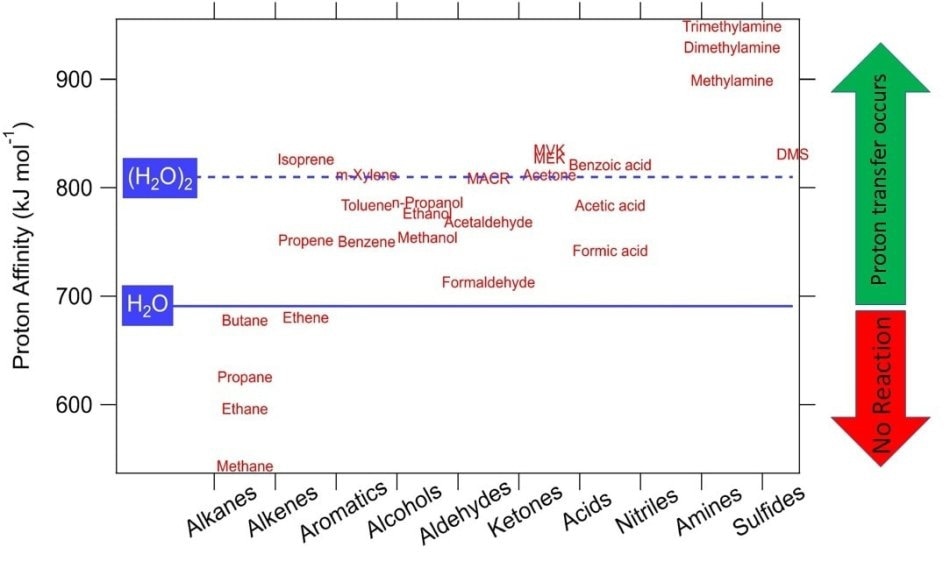
Figure 1. Proton affinities of some common VOCs. Compounds having higher proton affinity than water undergo proton transfer reactions with H3O+ and are detectable in real time with PTR-MS.
One major benefit of PTR-MS is that standard air constituents (O2, N2) exhibit lower proton affinity compared to water and do not react; this eliminates the need for diluting a buffer gas.
Instead of H3O+, other reagent ions can also be used with the PTR-MS instrument. NO+ and O2+ are standard examples, but other ions can also be used. This potential extends the array of compounds that can be identified to include VOCs exhibiting a proton affinity lower than that of water. Isomeric compounds — molecules containing same atoms but having a varied conformation — can be separated by rapidly switching between reagent ions. These compounds react differently with the numerous reagent ions.
The Main Components of a Proton Transfer Reaction Mass Spectrometer
Sampling Inlet
The gas-phase analyte molecules are drawn directly into the proton transfer reaction cell by the sampling inlet. Since inlet designs differ, factors like flow rate and inertness of materials should be considered. The inlet line can also be heated to enable the detection of “sticky” VOCs, and VOCs that are easily degraded by temperature can be analyzed using unheated inlets, which are typically preferred for this task.
Ion Source
The H3O+ reagent ions are produced in the ion source. A hollow cathode discharge is used by a standard design to generate the H3O+ ions from humidified air. Bogus ions — for example, O+, OH+, and H2O+ generated in the discharge besides H3O+ — undergo efficient reactions with the water that also result in the formation of H3O+ in such a way that the equilibrium ion population is extremely pure, comprising of 99.5% H3O+. The generated H3O+ ions are moved from the ion source to the proton transfer reaction cell by electric fields. The ions in the proton transfer reaction cell interact with gaseous analyte molecules coming out of the sampling inlet.
Proton Transfer Reaction Cell (Also Known as Drift Cell)
H3O+ ions react with VOCs within a volume that has controlled and stable electric fields, pressure, and temperature. This stability and tunability are vital to offer absolute and real-time quantification of VOC concentrations. The proton transfer reaction cell contains pressure that is usually of the order of 2 mbar. The chamber also contains electric fields, which shift the product ions (that is, the protonated VOCs) and reagent H3O+ ions along the “drift axis” toward the detector region.
Furthermore, the quantity E/N is easily used to detail the conditions in the reaction chamber, where N refers to the gas number density and E signifies the electric field along the drift axis. Usual E/N values are in the range of 80–50 Td. Traditional designs include just a linear, DC field along the drift axis. The TOFWERK Vocus reaction cell exclusively superimposes oscillating RF fields on top of this linear, DC field to raise the net transmission of ions out of the reaction chamber and thus enhance sensitivity.
Ion Focusing Interface
Ions should be efficiently transmitted from the proton transfer reaction cell to the mass analyzer by the Ion focusing interface, while the difference in pressure between the low-pressure mass analyzer (for example, 1E-6 mbar) and the reaction cell (for example, 2 mbar) should be simultaneously reconciled. The reduction in pressure from the reaction cell to the mass analyzer happens across a sequence of differentially pumped vacuum stages, each exhibiting lower pressure.
The ions are steered and focused through these stages using ion optics. Designs are variable and may include static steering and focusing elements as well as RF components like quadrupole ion guides. The achieved sensitivity can be considerably affected by the efficiency with which the interface is able to transfer the ions into the mass analyzer.
Mass Analyzer
A mass analyzer is used to detect analyte ions, allowing the identification of VOCs on the basis of their mass-to-charge ratio (m/Q) and enabling the determination of their concentrations on the basis of the recorded signal intensity. Quadrupole mass analyzers were used by the first PTR-MS instruments. These analyzers are filtering devices that permit ions of a single m/Q to hit the detector at any moment. Specific m/Q values of interest can be monitored by users, or even an entire range of m/Q can be scanned to create a complete mass spectrum.
Normally, quadrupole mass analyzers have unit mass resolution, which means they are incapable of resolving ions exhibiting the same nominal mass-to-charge ratio. Although several academic groups have examined the application of ion trap mass analyzers for PTR-MS, this has not been provided at a commercial scale.
Recently, the application of time-of-flight, TOF mass analyzers has become increasingly common. Ions are separated by TOF mass analyzers depending on the variations in their velocities following acceleration by a fixed potential. All mass-to-charge ratios are inherently measured by TOF mass analyzers at the same time, and thus excel for any PTR-MS analyses intending to quantify several different VOC species. Moreover, compared to quadrupole mass analyzers, TOF mass analyzers can have much higher mass resolving power. In comparison with commercially available PTR-MS, the TOFWERK Vocus PTR-TOF has the highest available mass resolving power and thus offers the potential to isolate several isobaric VOCs and also to derive the total formula of recorded compounds. In addition, TOF-based PTR-MS systems offer a better mass range (>1000 Th) and time resolution compared to quadrupole-based systems.
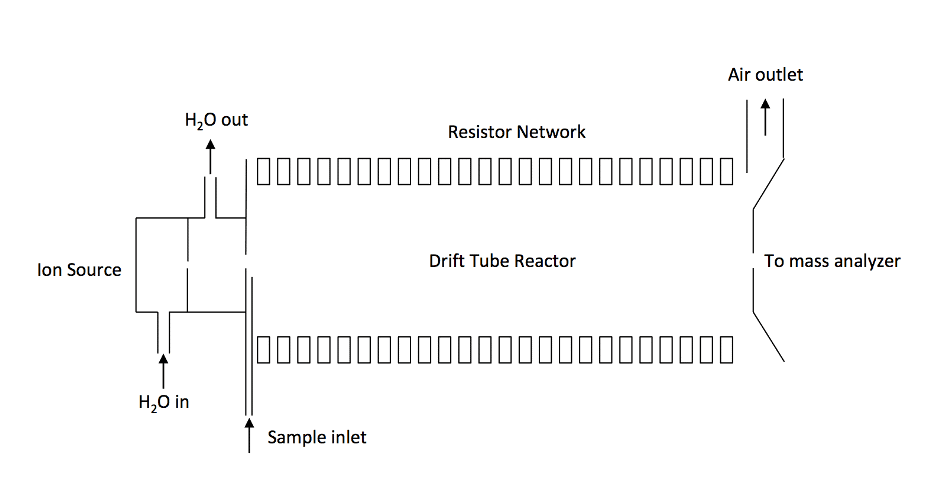
Figure 2. Schematics of a traditional PTR-MS instrument consisting of an ion source. An ion source gas port and a drift reactor. A distinguishing feature of the PTR-MS is that the drift tube is constructed by a series of ring electrodes.
A Historical Perspective of Proton Transfer Reaction Mass Spectrometry
PTR-MS has its origins in what is known as the “flowing afterglow” method, which Ferguson and coworkers pioneered in the 1960s for use in the analyses of ion-molecule reactions. This method involves the generation of ions through a discharge in a gas. The discharge’s bright glow extends from the ion source region into a neighboring region that contains a flow of inert buffer gas combined with trace amounts of neutral molecules. Within this afterglow, ions react with the neutral molecules, thus enabling the analyses of ion-molecule reactions.
The development of the selective ion flow tube, or SIFT, method2 in the 1970s was the first progression of the afterglow techniques. The SIFT technique can overcome a drawback of the original afterglow technique in which ions are not selected before reaction with the neutral molecules. In the SIFT technique, only ions of a specific mass-to-charge ratio are transmitted into the flow tube by a quadrupole filter to react with the neutral molecules.
Currently, SIFT is not only used for studying the reactions between ions and molecules but also used for examining trace gases in air. In the latter application, called SIFT-MS, the neutral molecules are the target analytes, and detection is made using a mass analyzer after the reaction flow tube reactor.
Introduced by Lindinger and coworkers3 in the 1990s, PTR-MS represents a further evolution. It adds two innovations. Instead of selecting reagent ions with a quadrupole, a hollow-cathode discharge generates the reagent ions with very high purity. Secondly, a relatively short drift tube replaces the flow tube, applying an electric field rather than a carrier gas to transmit ions. The overall result is a gain of several orders of magnitude in the detection sensitivity for VOCs when compared to the SIFT-MS technique.4
References
- Ferguson, E. E., Fehsenfeld, F. C. & Schmeltekopf, A. L. Flowing Afterglow Measurements of Ion-Neutral Reactions. In Advances in Atomic and Molecular Physics (ed. D.R. Bates and Immanuel Estermann) Volume 5, 1–56 (Academic Press, 1969).
- Adams, N. G. & Smith, D. The selected ion flow tube (SIFT); a technique for studying ion-neutral reactions. Int. J. Mass Spectrom. Ion Phys. 21, 349–359 (1976).
- Hansel, A. et al. Proton transfer reaction mass spectrometry: on-line trace gas analysis at the ppb level. Int. J. Mass Spectrom. Ion Process. 149–150, 609–619 (1995).
- Blake, R., Monks, P. & Ellis, A. Proton-Transfer Reaction Mass Spectrometry. Chem. Rev. 109, 861–896 (2009).

This information has been sourced, reviewed and adapted from materials provided by TOFWERK.
For more information on this source, please visit TOFWERK.