It is commonly understood that reducing oxides at a high H2 pressure notably reduces the reduction temperature,1 consequently minimizing or reducing the sintering of the active species dispersed on the solids’ surfaces.2,3,4,5
Everyone recognizes that growing particles is a requirement of sintering an active species. This reduces the active surface area where a reaction would normally occur. If the active area is reduced, the catalyst is less active.
In order to track the depletion of H2 during reduction, an Flow Reactor (FR) connected to an MKS Cirrus II mass spectrometer was used to perform numerous reduction experiments on the Micromeritics reference material of silver oxide (Ag2O).
Observations recorded that while a high pressure of the reducible mixture was used, there was a delayed response from the mass spectrometer which indicated the opposite result of what was anticipated. This means that as the reducible gas pressure increased, one would expect a lower reduction in temperature.
It makes sense to imagine that high pressures inside the reactor will cause delays in the effluent molecules getting out to reach the mass spectrometer. The reduction temperature displayed by the MS was, therefore, an artifact.
As a means of solving this issue, numerous tests were undertaken with a view to calibrating the MS signal and lowering the signal to the expected, normal value. As a reference for all of the tests, a flow rate of 50 ml per minute of the reducible mixture was used. Corresponding to carbon monoxide (CO), the mass spectrometer was set to follow a mass of 28.
The first test, which lasted roughly 630 seconds, involved injecting a pulse of approximately 0.5 ml of CO and observing the MS response. The subsequent step involved increasing the pressure to 5 bars while maintaining the same gas flow rate (50 ml per minute).
Once more, there was a pulse made of CO and the mass of 28 was followed. The response lasted 750 seconds. In order to synchronize the response time, the flow was increased to 250 ml per minute in order to produce the same response time of 630 seconds.
The above procedure was repeated for 10 bars and 20 bars with the corresponding flow rates being shown in Table 1. As the pressure rose, a shift of the reduction temperature was observed. It makes sense to imagine that the mixture’s higher flow rate and the high pressure directly influenced the reduction of the oxide.
It must, therefore, be concluded that, with a minimum sintering effect, a lower reduction temperature for a material positively impacts the reduction of the active species.
Table 1. This table shows the resulting reduction temperature before and after the adjustment made to the flow at each considered pressure.
Flow
ml/min |
Pressure
(bar) |
Reduction
Temp. (C) |
Response
Time (S) |
50
50
250 |
1
5
5 |
130
145
125 |
630
750
600 |
50
480 |
10
10 |
160
120 |
810
570 |
50
925 |
20
20 |
170
120 |
870
570 |
.jpg)
Figure 1. This figure shows the device used for these experiments as well as the double-headed thermocouple.
Experimental
The Flow Reactor (FR) was used to carry out all of the tests, with a 9 mm id SS straight tube reactor. For these experiments, a double-headed thermocouple was used, as depicted in Figure 1. One of the thermocouple’s heads was connected to the mass spectrometer and the other was used to control and heat the oven where the reactor was situated, in order to pick up both of the required reduction temperatures.
As the blue graphs show, an initial detected temperature which appeared on the temperature curve graph of the mass spectrometer was taken at the area of the curve at which the liberated heat from the exothermic reaction was located.
As the thermocouple was inserted in the catalyst bed, this temperature was deemed to be the actual reduction temperature. It is, therefore, able to detect any temperature fluctuations.
As the red graphs show, the second reduction temperature was taken at the zenith of the peak, which was detected by following mass 2 at the mass spectrometer.
This graph demonstrates the observed shift in temperature as it is detected when the hydrogen depletion signal gets to the instrument’s analysis chamber. The FR’s liquid gas separator, which has the ability to cool to temperatures between 3 °C and 4 °C, was used to trap the water produced during reduction.
In order to achieve the final reduction temperature, the oven’s temperature was ramped with a rate of 10 °C per minute. After the cold trap, the Cirrus II MKS mass spectrometer was connected to the reactor’s exhaust. During all of the reduction experiments, this was set to follow mass 2 for H2. It was set to mass 28 for the calibration purpose.
The Micromeritics MicroActive program was used to reduce all of the data. This enabled peaks to be integrated in addition to the maximum temperature of every peak being detected.
Results
This study’s results confirm the initial suppositions of the experiment – namely, that high pressures lead to a shift in the reduction temperature, which has to be corrected in order to obtain the precise reduction temperature.
Shown in Table 1 is the behavior of the reduction temperature of the silver oxide prior to the adjustments being made to the flow. As is seen from the experiments plotted in Figure 2, as the pressure increased, all of the reduction temperatures increased.
Figure 3 shows the expected reduction temperatures obtained once the flow has been adjusted in accordance with the calibration. All of the results obtained in this experiment indicated that as the pressure inside the reactor increases at the point at which the studied sample is situated, there is a shift in the reduction temperature as a result of the mass spectrometer’s delayed response.
.jpg)
Figure 2. This figure shows the apparent shift on the reduction temperature detected by the mass spectrometer as the pressure increases, compared to the basic reduction temperature of 130 C for Silver Oxide.
.jpg)
Figure 3. This figure shows the reduction temperature as detected by the mass spectrometer after adjusting the flow according to the calibration mentioned in the text.
Conclusion
This article has shown that the reduction temperature of any oxide sample is influenced by a high pressure of H2. As oxides reduce at lower temperatures, the active particles which are encountered on the surface do not undergo significant transformations. Consequently, the solid’s activity remains in optimum conditions.
However, if experiments are undertaken at higher hydrogen pressures, the response of the depleting hydrogen at the detection device will be delayed, potentially producing incorrect data. By increasing and calibrating the H2 flow, the expected results will be produced – namely, a lower reduction temperature as a function of increasing pressure over the oxide sample bed.
References and Further Reading
- Hydrogen Reduction of Germanium Dioxide under High Pressure* R.Haregawa, T. Kurosawa and T. Yagihashi. J. Japan Inst. Metals. 34 (1970), 481
- Analytical Methods in Fine Particle Technology. P. Webb and C. Orr. Micromeritics Inst. Corp. 1995.
- Reduction of CuO and Cu2O with H2 : H Embedding and Kinetic Effects in the Formation of suboxides. Jae Y. Kim,†José A. Rodriguez,*†Jonathan C. Hanson,†Anatoly I. Frenkel,§ andPeter L. Lee‡ . J. Am. Chem. Soc., 2003, 125 (35), pp 10684–1069
- On the Reduction of Copper Oxide. H.P.Wang and C.T.Yeh. J.Chinese Chem. Soc.,30, 139-143, (1983)
- Reduction of MgO-supported iron oxide: formation and characterization of Fe/MgO catalysts. G. Bond, K. C. Molloy and F.S. Stone. Solid State Ionics, Volumes 101–103, Part 2, November 1997, Pages 697-705
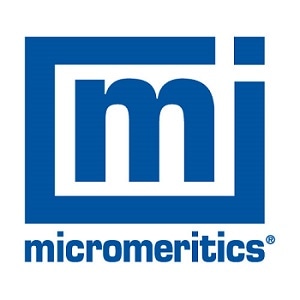
This information has been sourced, reviewed and adapted from materials provided by Micromeritics Instrument Corporation.
For more information on this source, please visit Micromeritics Instrument Corporation.