Despite whether one is manufacturing inexpensive vertical-cavity surface-emitting laser diodes (VCSELS) for consumer electronics or utilizing a tunable mode-locked Ti:Sapphire laser for multi-photon fluorescence microscopy, having correct spectral data throughout the manufacturing procedure is essential for creating a high-quality and repeatable product.
Regrettably, a vast number of laser engineers continue to rely solely on large and expensive optical spectrum analyzers (OSAs) for spectral testing. Although these devices give outstanding dynamic range and spectral resolution, the cost and size frequently reduce their ability to be utilized at several points within the environment of manufacturing [1].
Moreover, very low sensitivity is a result of the scanning nature of these devices, which leads to lengthy integration times, making OSAs incompatible with high-speed automated production lines [1].
As an alternative, miniature spectrometers benefit from a fixed grating spectrograph in conjunction with a linear detector array to allow the whole spectrum to be analyzed at the same time, and at high speeds.
Although this geometry does not provide real-time adjustment of spectral resolution and range, it does provide much greater sensitivity in comparison to the scanning geometry utilized in OSAs as it does not need a different acquisition at each point of measurement.
The advantage of speed is not only beneficial in fast-paced high-volume testing environments but also provides accurate measuring of pulsed laser systems by employing high-speed triggering. As a consequence, certain manufacturers of lasers are using miniature spectrometer technology as a complementary technology to the more common OSA.
.jpg)
This article will outline the advantages of miniature spectrometers for laser characterization in-line along with in the laboratory. It is critical to firstly explore the bigger picture and briefly review the fundamentals of miniature spectrometers.
Fundamentals of Miniature Spectrometers
Similar to all spectrometers, Avantes’ miniature spectrometers are fundamentally an imaging system that divides the image of the entrance slit into a vast number of spatially separated, monochromatic images in the detection plane.
In the Czerny-Turner spectrograph design, this is achieved by initially collimating the light from the entrance slit utilizing a concave mirror and guiding it to a reflective dispersion grating. The various wavelengths of light consequently reflects from the grating at different angles, spatially separating more and more as they travel further from the grating.
This variety of collimated rays is then directed back down to produce an image of the entrance slit at the detector plane by a bigger truncated focusing mirror, but due to their angular separation, the image of each wavelength is now divided along the axis perpendicular to the location of the entrance slit.
By putting a linear array in the image plane, every pixel can be calibrated to adhere to a distinct wavelength. Figure 1 below illustrates a schematic diagram for a common Czerny-Turner miniature spectrometer.
.jpg)
Figure 1. Typical Avantes spectrometer with the beam path through the Czerny-Turner spectrograph to the detector overlaid.
It is essential to note that because of the off-axis nature of the Czerny-Turner optical design, at low f-numbers, these systems typically exhibit high amounts of coma†, which can cause asymmetric peak distortions and a decreased spectral resolution.
Due to this, all of Avantes’ high-resolution miniature spectrometers for laser testing are manufactured with a large f-number because throughput is much less important than resolution for laser measurement.
It must also be noted, however, that it is important to fill the complete numerical aperture of the collimating mirror when employing this specific spectrograph for laser testing.
This instruction may seem obvious but if a laser is directly aimed into the entrance slit it will not be properly collimated and as such will not strike the grating at the correct angle and with the accurate fill factor, which will create erroneous artifacts in the spectrum.
This error will cause a single-mode laser to appear to have several spectral modes; as such, it is always advised that the laser light is coupled into the spectrometer through a fiber optic cable to maintain the accuracy of the spectral measurement.
† Coma is an off-axis optical aberration that makes the image blur out and have a tail, appearing like an ice cream cone or a comet. This decrease in the quality of the image is inversely proportional to the f-number of the optical system creating a tradeoff between optical throughput and image quality.
Laser Testing Configuration
When setting up a miniature spectrometer, three major components, the entrance slit, detector array, and dispersion grating, needs to be specified up front as these function simultaneously to configure the spectral range, sensitivity, and resolution of the instrument.
For laser characterization where an excess of spectral energy density exists, it is always recommendable to configure these options to give the highest dynamic range and resolution, because spectral range and sensitivity are much less important.
This is commonly achieved by using a CMOS detector array with the smallest pixel pitch and slit size possible, and a high groove density grating.
From the spectrograph geometry, it is obvious that this selection of pixel size, slit width, and groove density will all result in the narrowest achievable resolution, but it is not as obvious why a CMOS array would be preferred over a CCD array.
When compared to CCD detectors, CMOS detectors tend to provide larger dynamic ranges and an increased linear responsivity, which is very important for the detection of small variations in large signals.
To show this effect, an 830 nm laser from Innovative Photonic Solutions model number I0830MB0600M-HP was tested utilizing an AvaSpec-ULS468CL-EVO) with a 5 mm entrance slit and a 1,200 groove/mm grating.
The spectrometer was coupled using an SMA905 fiber patch cord to a cosine corrector and irradiance calibrated, to convert the output from arbitrary counts per nanometer to spectral irradiance, as demonstrated in Figure 2A.
.png)
Figure 2. (A) 830 nm laser spectra as drive current increases from 250 mA to 550 mA, (B) linear regression model based on peak spectral irradiance, and (C) linear regression model for integrated laser power each of which produces an R2 > 0.99.
It is essential to highlight that the output of this laser was coupled to a 0.22NA 105 mm fiber optical cable and finished at an SMA905 bulkhead creating a homogenized divergent output beam. The cosine corrector was fixed at a set distance in front of the diverging beam which uniformly illuminated it, as the drive current was changed using the lasers USB interface.
This configuration was utilized to stop the spectrometer from saturating while the test was running, but as a consequence of this, the total laser power was incalculable.
Rather, the power incident on the active area of the cosine corrector was analyzed and is presented in Figure 2C, by numerical integration of the spectra and the multiplication of the result by the active area of the cosine corrector.
The data displayed in Figure 2 outlines the incredibly high linearity of the CMOS detector with an R2 > 0.99, including the integrated laser power and peak spectral irradiance. An integrating sphere must be utilized instead of a cosine corrector to analyze the total output power from such a high-power laser.
Application Examples
Now that the standard requirements for laser testing with miniature spectrometers has been thoroughly outlined, this article will go on to illustrate a few common examples demonstrating where they are the preferred choice over OSAs.
The next section will assess how Avantes’ spectrometers are employed in two separate applications: tunable laser monitoring and VCSEL manufacturing. While not an exhaustive list, it delivers a strong outline of the benefits of applying the technology
Avantes Spectrometers in VCSEL Manufacturing and Testing
VCSELs can be measured at different stages of production, in contrast to edge-emitting laser diodes which cannot be tested before the die are cut from the wafer. This includes whole wafer testing as the laser light is emitted perpendicular to the pn-junction [2].
This supports the testing of thousands of VCSELs at the same time and on a single wafer, resulting in more efficient production and decreased scrap rates. Consequently, high-speed spectral measurement on the production line is necessary to utilize the distinctive wafer level testing ability exclusive to VCSELs.
Device manufacturers and integrators need optical spectrometers that feature a high resolution, short integration time, and a high speed triggering response, all of which can simply be provided by Avantes’ miniature spectrometers.
Figure 3 demonstrates three different examples of VCSEL spectra gathered through wafer processing. Figure 3A is an example of a single-mode VSCEL which is correctly manufactured showing only one peak, while Figure 3B shows a faulty single mode VSCEL with an undesirable sidemode.
Through the incorporation of high-speed spectrometers into the wafer inspection process, VCSEL manufacturers can now use sorting technology and high-speed binning close to what is utilized in the LED manufacturing process to dramatically lessen costs.
.png)
Figure 3. Example VCSEL spectral profile demonstrating a correctly functioning single-mode laser output (A) and a malfunctioning laser with a large side mode (B).
Avantes Spectrometers in Tunable Laser Monitoring
A high number of typical laboratory laser systems can all contain tunable outputs such as external cavity lasers (ECLs), mode-locked Ti:Sapphire lasers, and optical parametric oscillators (OPOs). When handling these tunable wavelength laser sources, it is often the case that the monitoring output does not need the precision of an OSA.
This is particularly true when using picosecond to femtosecond pulse width lasers††, where the spectral bandwidth can frequently vary between 0.5 nm and 20 nm. For example, Figure 4 below demonstrates the spectrum of a 221 fs pulse width tunable Ti:Sapphire laser system at 800 nm, providing a tuning range of around 170 nm [3].
.png)
Figure 4. Spectral profile of a 221 fs pulse width Ti:Sapphire tunable laser [3].
Due to this reason, miniature spectrometers for laser testing are not only applicable within a production environment. Cost-efficient miniature spectrometers, for example the AvaSpec-Mini 4096CL can be configured with a spectral resolution as fine as 0.5 nm, and are perfect for observing the tuning of these ultra-fast lasers in a laboratory setting.
For more complex applications, for example monitoring ECLs, another tunable narrow linewidth laser source such as the AvaSpec-ULS4096CL, with a resolution smaller than 0.1 nm, is a great alternative to OSAs.
Avantes instruments are utilized to assist several pulsed laser applications along with tunable laser monitoring due to their exceptional timing control electronics. When profiling a pulsed laser in a repeatable manner, the spectrometer utilized must allow for external triggering and provide an almost instant response upon receiving a trigger.
Avantes instruments use an on-board field-programmable gate array (FPGA) to command the timing performance, providing low-jitter, high-speed timing controls with programmable external trigger delays as quick as 870 ns with jitter of 21 ns.
†† To clarify, it must be noted that in the case of ultra-fast laser measurement, the spectrometer is not analyzing a single pulse, but rather integrates over a high number of pulses measuring the average spectral bandwidth throughout measurement.
Conclusion
As outlined in this article, many customers consider Avantes instruments to provide beneficial alternatives for bigger and more expensive OSAs for most of their laser testing needs.
The new Mini4096CL, along with the AvaSpec-ULS4096CL-EVO and the AvaSpec ULS3648-USB2 are the three most frequently utilized for laser characterization by various laser integrators and manufacturers in adherence to their in-line quality control procedures.
These miniature spectrometers are employed to analyze a range of common laser parameters such as centroid, spectral peak wavelength, Full Width Half Maximum (FWHM), side mode suppression ratio, and RMS spectral bandwidth.
These parameters can be specifically measured utilizing the AvaSoft software interface, or users can deploy Avantes’ easy to implement DLL library which makes integration with production software convenient.
Related Products
.jpg)
AVASPEC-ULS4096CL-EVO
.jpg)
AVASPEC-MINI4096CL
.jpg)
AVASPEC-ULS3648
.jpg)
AVASPEC-ULS2048
The simple software integration, high-resolution, high-sensitivity and high-speed acquisition features of the AvaSpec range of spectrometers makes them a cost-effective alternative to OSAs for a wide subset of laser characterization and testing.
By using fiber-coupled optical sampling accessories, including integrating spheres and cosine correctors, Avantes’ spectrometers can characterize all types of lasers regardless of output power and beam divergence.
To find out why so many laser scientists and engineers are looking at Avantes for trustworthy equipment, contact the dedicated application support specialists today.
References and Further Reading
- V. Coffey, “Optical Spectrum Analyzers: Understanding the latest features in optical spectrum analyzers,” Laser Focus World, June 2010.
- K. Iga, “Surface-Emitting Laser—Its Birth and Generation of New Optoelectronics Field” IEEE Journal Selected Topics in Quantum Electronics, Vol. 6, No. 6, November/December 2000.
- P.G. Antal and R. Szipocs, “Tunable, low-repetition-rate, cost-efficient femtosecond Ti:sapphire laser for nonlinear microscopy,” Appl Phys B (2012) 107:17–22
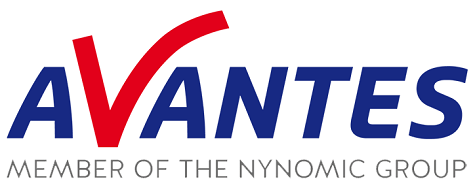
This information has been sourced, reviewed and adapted from materials provided by Avantes BV.
For more information on this source, please visit Avantes BV.