Mercury is thought to be one of the top ten chemicals of public health concern by the World Health Organization. It is a neurotoxin that, in small quantities, quickly bioaccumulates and can cause major health problems or even death.
Chronic exposure at low levels is known to affect the digestive, nervous, and immune systems, in addition to the kidneys, lungs, and eyes with breastfeeding babies and fetuses susceptible to developmental effects.
Found in water, air, and soil through volcanic activity, mercury is a naturally occurring element. However, human activity significantly contributes through waste incineration, the burning of coal, mining and other industrial uses, finding its way into the environment and eventually, into the food chain.
Mercury exists in several forms, including the inorganic form as in mercury salts, the elemental or metallic form, and the organic form with methylmercury being the most toxic. Establishing mercury concentrations in environmental samples such as wastewaters and natural waters is crucial in environmental risk assessment.
The “Directive 2000/60/EC of the European Parliament and of the Council established a framework for the Community action in the field of water policy” or, in short, the EU Water Framework Directive was formerly adopted on the 23rd of October 2000. Mercury and its compounds are part of this directive.
Environmental quality standards (EQS) of 2008/105/EC define the maximum concentration (MAC) for mercury permitted as 0.05 µg/L. The limits of quantification for mercury in environmental waters are set in recent regulations. These limits can be seen in table 1.
Table 1. Limits of quantification relative to Hg.
Code |
Name |
Matrix |
Limit of quantification |
Effective date |
1387 |
Mercury |
Freshwater |
0.050 µg/L |
Prior to the 15th of July 2013 |
1387 |
Mercury |
Freshwater |
0.015 µg/L |
From the 15th of July 2013 |
1387 |
Mercury |
Wastewater |
0.5 µg/l |
From the 15th of July 2013 |
Instrumentation
- ASPQ 3300 autosampler
- ESI oneFAST high-throughput sample introduction system
- PlasmaQuant® MS: Inductively Coupled Plasma – Mass Spectrometer
Method
The system was optimized for lowest detection limits of minimum oxide formation and mercury. For maximum signal for Hg isotopes listed in table 2, a solution of 1 µg/L Hg was utilized to optimize the ion optics.
For calculations, the sum of the four Hg isotope masses were utilized, enabling a higher signal to be recorded for better precision and detection limits.
The plasma conditions were set for minimum oxide formation at <2% (CeO to Ce ratio), As there is the potential for Hg isotopes to be interfered with by the formation of tungsten oxides (WO+) polyatomics. The instrument parameters are shown in table 2.
Table 2. Instrument parameters.
Parameter |
Specification |
Plasma Gas |
9.0 L/min |
Auxiliary Gas |
1.25 L/min |
Nebulization Gas |
1.00 L/min |
Sampling Depth |
5.0 mm |
RF power |
1.4 kW |
Pump rate |
10 rpm |
Measured Hg isotopes |
198, 199, 200 and 202 |
Scan Mode |
Peak hopping |
Points per Peak |
1 |
Scans per Replicate |
30 |
Replicates |
3 |
Dwell Time |
50 ms |
Rinse time |
30 s |
Samples and Reagents
- Mono-elemental mercury stock standard solution (100 mg/L, Inorganic Ventures)
- Potassium bromate (Merck)
- High purity ICP-MS grade nitric acid (NORMATOM from VWR)
- Potassium bromide (Merck)
- High purity ICP-MS grade hydrochloric acid (NORMATOM from VWR)
- LGC Standards ERM-CA615 Ground water (Certified Reference Material)
- National Research Council Canada ORMS-5 River Water (Certified Reference Material)
- Hydroxylammonium chloride (Merck).
Sample and Standard Preparation
A combined step of conservation and digestion was performed. By utilizing a mixture of potassium bromide and potassium bromate (KBr/KBrO3) in 1% HCl, mono and organomercury compounds of Hg are converted into divalent mercury by oxidation.
Hydroxyl ammonium chloride is used to stabilize the excess of bromide. Around 5.95 g of KBr (Merck) and 1.39 g of KBrO3 (Merck) were dissolved in 500 ml deionized water. Prior to dissolution, both powders (KBr and KBrO3) were dried overnight at 300 °C to remove any traces of mercury contamination.
The hydroxyl ammonium chloride (NH4ClOH) solution was prepared by dissolving 6 g in 50 ml of deionized water. Solutions were prepared by adding 0.5 mL of 1% HCl (v/v) and 1.0 mL of the above KBr/KBrO3 solution into 50 mL of the standard and sample solutions.
Next, they were left at room temperature for 15 minutes to enable the reaction to happen (the solutions develop a yellow tint) and then to neutralize the Br2 formed, 1.0 mL of NH4ClOH was added to produce a clear solution. Three proficiency test water samples and two certified water reference materials were prepared and analyzed.
To make up all the calibration solutions, a mono elemental mercury stock standard solution from Inorganic Ventures (100 mg/L) was utilized, covering the range from 10 ng/L up to 1000 ng/L (figure 1). To correct for any possible signal drift or matrix effect, 193Ir was used as an internal standard.
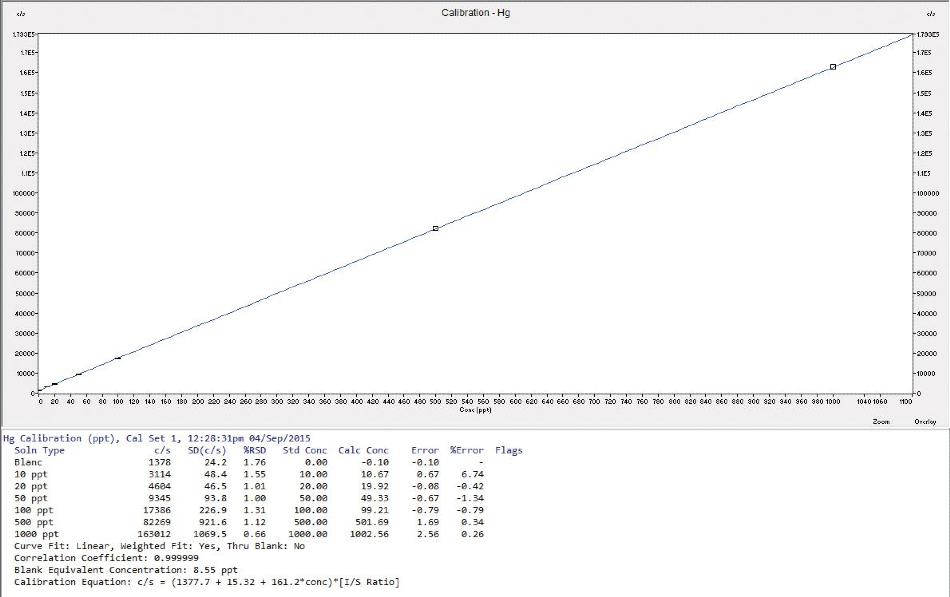
Figure 1. Calibration curve from the summation of Hg 199-202 isotopes.
Results and Discussion
Table 3 shows the measured and certified values for Hg in river and groundwater reference materials. The measured and expected values for drinking, natural, and waste waters, together with the Z-score are shown in table 4.
Table 3. Reference materials results.
Reference material |
Certified value (ng/L) |
Measured value (ng/L) |
ORMS-5 River water |
37.0 ± 4.0 |
36.6 ± 0.8 |
ERM-CA615 Groundwater |
26.2 ± 1.3 |
26.4 ± 0.8 |
Table 4. Proficiency tests results.
Proficiency test |
Measured value (µg/L) |
Assigned value (µg/L) |
Z-score |
Natural water |
1.2 |
1.1 |
0.3 |
Drinking water |
2.7 |
2.5 |
1.0 |
Waste water |
34.5 |
35.0 |
-0.1 |
The Z-score shows how many standard deviations the measured value is from the mean value and for this analysis, was established to be ≤±1. Limit of quantification, linearity tests, and accuracy profiles were assessed according to the French norm NF T 90210 (2009).
Gathered from a river in Vendée, France, a natural water sample was utilized to estimate the limit of quantification (LOQ). A final LOQ of 2 ng/L was established together with a 3σ method limit of detection (MDL) of 0.3 ng/L.
The readback of a 2 ng/L spiked water sample measured 10 times over the duration of 5 days is shown in Figure 2. The readback at the LOQ was well within the ±60% concentration range as established by the NF T 90210 norm.
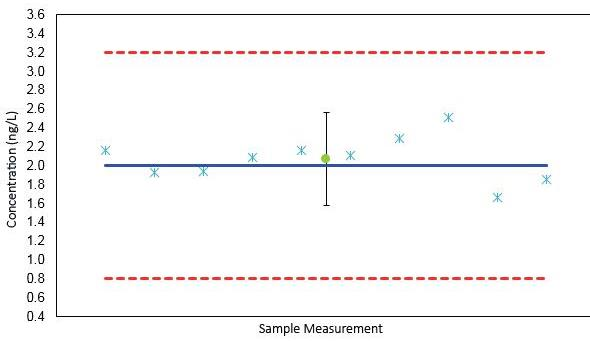
Figure 2. Validation of the LOQ according to the norm NF T 90210.
Sample Introduction Washout Test
Mercury is known to have serious memory effects, influencing data quality at ultra-trace concentrations. Instrument setup and sample preparation are crucial to minimize memory effects.
A study was performed to show the effectiveness of the sample preparation and the washout efficiency of the sample introduction system. The oneFAST high throughput sample introduction system from Elemental Scientific is a software-controlled, switching valve system that enhances the efficiency of sample uptake and washout massively.
This includes decreasing the volume of sample needed for analysis and contact time with sample introduction tubing. A blank solution was routinely run straight after the top standard (1000 ng/L) with washout to less than 1 ng/L attained in less than 30 seconds.
Conclusion
Reliable ultra-trace determination of mercury in environmental waters is provided by the superior sensitivity of the PlasmaQuant® MS. A method LOD of 0.3 ng/L was gathered without the requirement for pre-concentration methods (e.g. hydride or cold vapor generation).
The ASPQ 3300 autosampler with oneFAST sample introduction system ensures reduced memory effects and high efficiency. Results which are gathered on proficiency tests and reference materials were well within defined ranges and in excellent agreement with certified values.
The modern RF generator design of the PlasmaQuant® MS Elite supplies very robust plasma conditions for complex wastewater samples that contain high total dissolved solids, and with only half the argon gas consumption.
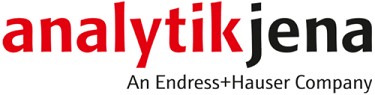
This information has been sourced, reviewed and adapted from materials provided by Analytik Jena US.
For more information on this source, please visit Analytik Jena US.