Sponsored by Mo-SciReviewed by Louis CastelFeb 21 2024
Glass, a highly functional material integral to modern technology, plays an active role in the world of electronics and energy. From the screens of our smartphones to the fibers that carry internet signals across oceans, glass is the backbone of many technologies that rely on its unique electrical properties.
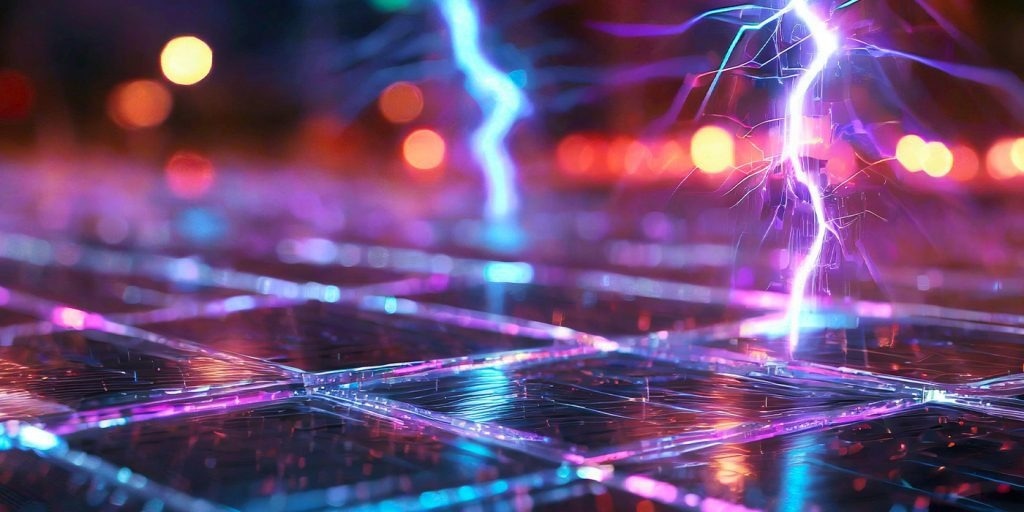
Image Credit: Mo-Sci
This article will outline some of these properties, ranging from dielectric constants to electrical resistivity while exploring how glass composition can impact each area.
We will also delve into the science behind these properties and their diverse applications in today’s technology-driven world. This journey will reveal not just a material but a versatile and essential component of countless electrical and electronic innovations.
The Relationship Between Composition and Electrical Properties
Glass was first selected for its insulating capacity in telegraph communications around 1845. Fast forward to the present day, and there are over 360,000 different compositions of glass documented.
Each composition has been specifically engineered with particular electrical, mechanical, and thermal properties in mind to meet an extensive range of ever-growing technological needs. This is a testament to the material’s versatility in electrical applications.
In this article, many of the commonly measured electrical properties of glass will be detailed,2 along with an examination of the effects of compositional variations on these properties. The aim of this article is to provide insight and show how different components play a role in enhancing or moderating these properties in high-tech applications.
The Dielectric Constant
The dielectric constant is a key factor in determining a material’s capacity to store electrical charge when exposed to an electric field. As a dielectric material, glass acts as an electrical insulator, meaning electrical charges are not able to flow through it as they do in conductor materials like metals.
Instead, when an electric field is applied, a displacement of the positive and negative charges within the glass occurs; positive charges are attracted towards the field, while negative charges are repelled away. This charge separation, or polarization, allows the glass to store charge as it limits the internal electric field in the glass.3
This dielectric behavior can be observed when glass is inserted between the plates of a parallel-plate capacitor. This directly influences the capacitance value, boosting its capacity to store opposing charges on each plate.3
The relationship between the dielectric constant and composition of glass is an ongoing research area. This is a complex relationship that requires a good understanding of how the different elemental components within glass contribute to polarization.
In general, glasses with greater dielectric constants typically contain alkalis, alkaline earth, and heavy-metal cations such as lead, bismuth, and tungsten.4 Research has made it clear that augmenting the composition of glass can have a bearing on its dielectric constant.
For example, the dielectric constant can be increased by substituting silicon for aluminum in sodium aluminosilicate glasses, raising tungsten oxide levels in sodium germanium borosilicate glasses, and modifying copper oxide levels in borate zinc-fluoride glasses. Conversely, introducing rare earth oxides to alkali-free aluminoborosilicate glass can reduce its dielectric constant.4,5,6,7
By altering the elemental makeup of glass, its electrical characteristics can be tailored to meet specific technological needs, paving the way for advanced and specialized uses in various industries.
Loss Tangent
When exposing glass to an alternating electric field, some of the electrical energy is stored in accordance with its dielectric constant. However, not all of the energy is stored efficiently; some is unavoidably lost, largely as heat, a phenomenon known as dielectric loss.
The dielectric loss tangent of glass is linked to this dissipation of electrical energy and is caused by a variety of physical processes, including but not limited to dielectric relaxation, dielectric resonance, and electrical conduction.8,9
For applications such as high-frequency electronic circuits, minimizing energy dissipation is vital. Therefore, the glass composition must be carefully tailored to achieve a low-loss tangent. This adjustment is akin to precision engineering, which ensures that the glass performs optimally, preventing any unnecessary energy wastage.
Researchers have observed that the levels of impurities in glass can influence dielectric loss. In the case of borosilicate (BRS) glass-reinforced PTFE composites used for microwave substrate applications, it was found that a decrease in BRS filler size can lead to a reduction in the loss tangent.10,11
This underscores the important role that material composition and structure play in achieving the required electrical properties for specific technological applications.
Conductivity and Resistivity
Conductivity and resistivity are crucial properties for many materials, particularly in relation to electronic applications. In simple terms, electronic conductivity entails the transmission of electrical charge by electrons, which are negatively or positively charged electron holes.
Glass is ideal for insulating applications as it demonstrates conductivity levels far lower than those in metals due to its covalent and ionic bonding structure.
Notably, two glass families demonstrate considerable electronic conduction properties:
- Oxide glasses comprised of high amounts of transition metal oxides show enhanced conductivity. These oxides are able to introduce unpaired electrons, which facilitates electron flow.
- Chalcogenide and tetrahedral glasses, where the intrinsic covalent bonding supports their conductive properties.12
By modifying certain elements within the glass, such as introducing elements with free electrons or adjusting the bonding structures, manufacturers are able to fabricate glass with specific characteristics that influence its conductivity and resistivity.
The capacity to customize glass properties in this way makes it a versatile material for a variety of electronic applications, from insulators to conductive components.
Applications
Glasses demonstrating low dielectric constants are key for applications that aim to limit electrical losses, signal distortion, or interference. For this reason, they find application in high-performance microelectronic systems, such as substrates in thick-film resistors and semiconductor packaging.
In contrast, glasses possessing high dielectric constants are crucial for use in high-magnitude capacitors and multilayer dielectrics.4,14
The ability to manipulate electronic conductivity properties in glass has also paved the way for many new opportunities in modern technology. In particular, the photoconductivity of selenium and arsenic-selenium glasses has been at the heart of radically transforming the photocopying process.
Furthermore, certain glasses that exhibit electronic conductivity can actively switch between insulator and semiconductor states. This novel property has found application in the development of computer memory devices, demonstrating how versatile glass is in electronic applications.
The photovoltaic characteristics of amorphous hydrogenated silicon, a product of its electronic conduction, also form the cornerstone of solar cell technology.12
Propelled by ongoing research, the applications of glass with unique electrical properties are continually expanding. Such advancements underscore the key role that the electronic conductivity of glass takes up across a number of high-tech industries. This showcases how important it is when it comes to shaping modern technology.
How can Mo-Sci help?
Meticulous calibration and consideration of glass composition are crucial for accurate and meaningful measurements of the aforementioned electrical properties.
Mo-Sci specializes in creating high-performance sealing glasses that are ideal for a wide range of applications. Our expertise extends to customizing solutions, including the production of specialized glass substrates and ultra-pure glass frit.
References and Further Reading
- Chen, J., et al. (2017) Generation of shock lamellae and melting in rocks by lightning-induced shock waves and electrical heating. Geophysical Research Letters. doi.org/10.1002/2017GL073843
- Jones, R. (2010) An Incomplete History and Timeline of the Electric Telegraph and the CD 731 Compromise Insulator. Available at: https://www.insulators.info/shows/handouts/cd_731.pdf
- Al-Amoudi, M.A. (2020) Determining Dielectric Constants of Glass and Thin Film Using a Parallel Plate Capacitor. International Journal of Applied Science and Engineering Review. ISSN: 2582-6271.
- Hsieh, C., et al. (1996) Correlation between dielectric constant and chemical structure of sodium silicate glasses. Journal of Applied Physics. doi.org/10.1063/1.363824
- Mundher, M. (2023) Tungsten oxide effects on conductivity, dielectric parameters, and density of sodium germanium borosilicate glass. Journal of Materials Science: Materials in Electronics. doi.org/10.1007/s10854-023-10280-6
- Shaaban, S.M., et al. (2023) Influence of Copper Ions on the Structural, Mechanical, Radiation Shielding and Dielectric Properties of Borate Zinc-Fluoride Glasses. Journal of Electronic Materials. doi.org/10.1007/s11664-023-10564-x
- Zhang, L., et al. (2020) Influence of rare earth oxides on structure, dielectric properties and viscosity of alkali-free aluminoborosilicate glasses. Journal of Non-Crystalline Solids. doi.org/10.1016/j.jnoncrysol.2020.119886
- Poplavko, Y.M. (2019) Chapter 7 – Dielectrics. Electronic Materials: Principles and Applied Science. doi.org/10.1016/B978-0-12-815780-0.00007-4
- Sebastian, M.T. (2008) Chapter Two – Measurement of Microwave Dielectric Properties and Factors Affecting Them. Dielectric Materials for Wireless Communication. doi.org/10.1016/B978-0-08-045330-9.00002-9
- Rodriguez-Cano, R., et al. (2023) Broadband Characterization of Silicate Materials for Potential 5G/6G Applications. IEEE Transactions on Instrumentation and Measurement. doi.org/10.1109/TIM.2023.3256463
- Alhaji, I.A., et al. (2021) Effects of Particle Size on the Dielectric, Mechanical, and Thermal Properties of Recycled Borosilicate Glass-Filled PTFE Microwave Substrates. Polymers. doi.org/10.3390/polym13152449
- Varshneya, A.K., et al. (2019) Chapter 16 – Electronic Conduction. Fundamentals of Inorganic Glasses (Third Edition). doi.org/10.1016/B978-0-12-816225-5.00016-X
- Mo Sci. (2022) Applications of Thin and Thick Glass Films. Available at: https://mo-sci.com/applications-of-thin-and-thick-glass-films/ (Accessed on 17 November 2023).
- Li, J.C., et al. (2012) Characterization of Semiconductor Surface Conductivity by Using Microscopic Four-Point Probe Technique. Physics Procedia. doi.org/10.1016/j.phpro.2012.03.568
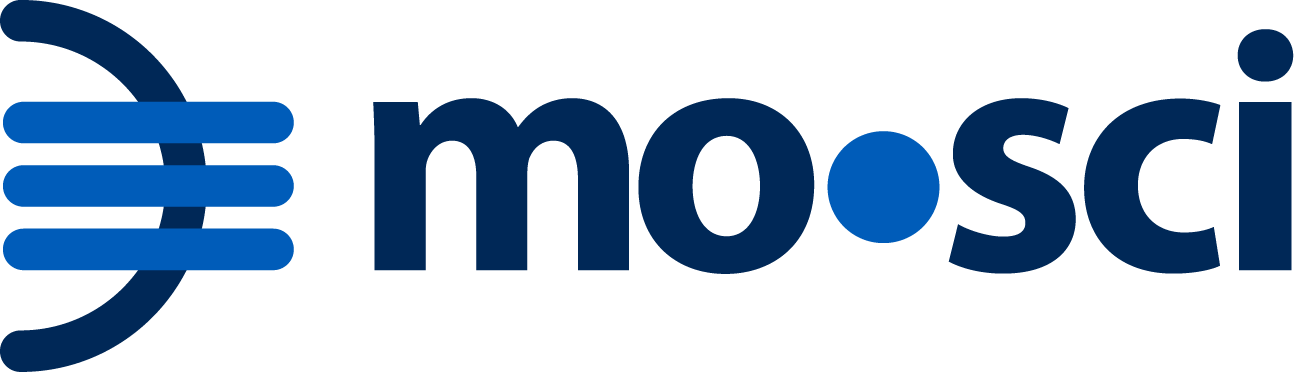
This information has been sourced, reviewed and adapted from materials provided by Mo-Sci.
For more information on this source, please visit Mo-Sci.