By Surbhi JainReviewed by Susha Cheriyedath, M.Sc.Sep 9 2022
In a review recently published in the open-access journal Materials, researchers discussed the recent development in the prevention of corrosion of degradable magnesium (Mg) for implant applications.
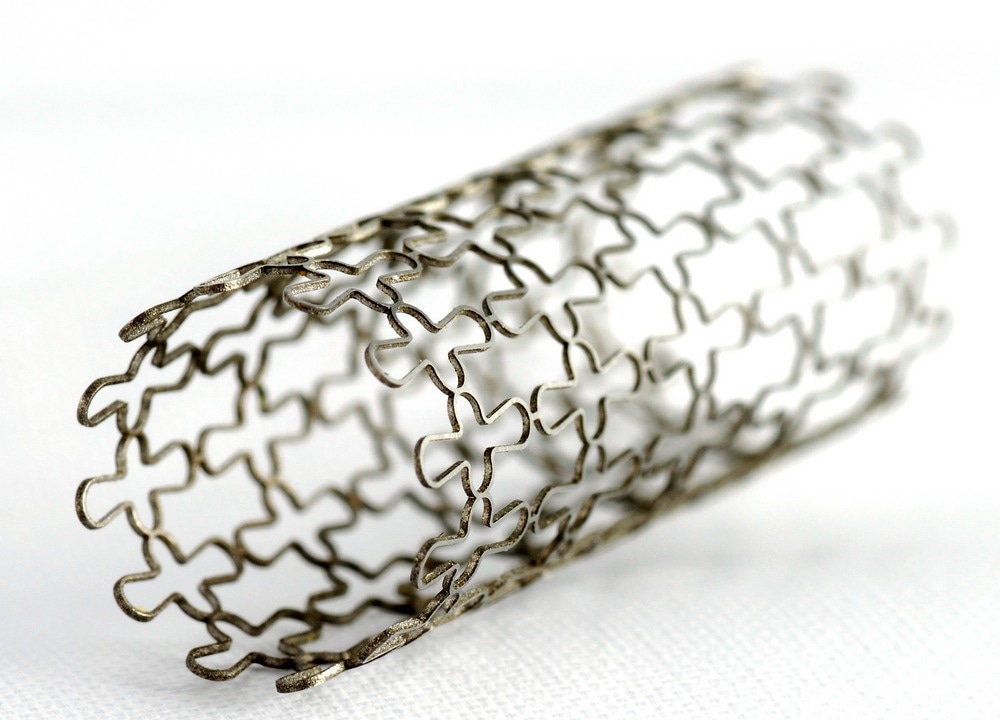
Study: Review: Degradable Magnesium Corrosion Control for Implant Applications. Image Credits: Floris Slooff/Shutterstock.com
Background
Due to their good machinability, high specific strength, and similar young's modulus to human bone, magnesium and its alloy can be employed as both a vascular stent and an ideal material for bone plates. Additionally, Mg is a crucial trace element involved in the entire metabolic process and is crucial for maintaining the biological function of the human enzyme system.
Mg can gradually decay during stent placement to prevent the development of vascular restenosis and the need for a second removal procedure. Patients experience less financial burden and surgical risk as well. However, at present, there are still several problems in Mg biomedical materials, restricting their wide applicability.
Since Mg and its alloys have a low standard electrode potential, they have poor corrosion resistance and are easily corroded in environments containing people. A faster rate of corrosion may cause mechanical instability loss and early failure of biomedical devices made of magnesium alloy before they can be used. The quick pace of degradation will also cause a significant amount of hydrogen to be generated in the human body.
Emphysema and localized alkalinity of the internal environment will develop if the hydrogen could not be released in a timely manner. Therefore, a pressing issue in recent years has been locating biomedical Mg alloys with strong biodegradability to satisfy the service requirements.
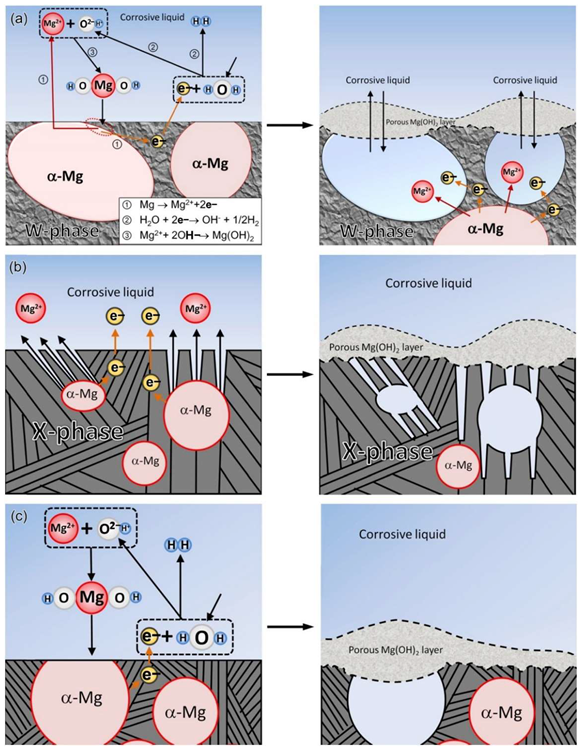
Schematic diagram of the corrosion mechanism: (a) MgY1.72Zn2.81Zr0.17 alloy, (b) MgY3.83Zn3.03Zr0.17 alloy, (c) MgY2.58Zn1.27Zr0.15 alloy. Image Credit: Wang, L et al., Materials
About the Study
In this study, the authors discussed the utility of Mg as biomaterials during the past 20 years and their associated high biological compatibility. The significance of controlling the corrosion resistance of magnesium alloys owing to their relatively low corrosion resistance for use in degradable implant applications was illustrated. The relative corrosion mechanisms of magnesium alloys, such as pitting, filiform, stress corrosion, high temperature, etc., were demonstrated.
The team outlined numerous techniques such as purification, micro-alloying, grain refinement, and surface modifications for the regulation of corrosion and biological performance. Additionally, the in vivo implantations of Mg alloy vascular stents were discussed, as well as the problems that were reported in recent years.
The researchers stated that to prevent unnecessary compounds, the concentration of micro-alloy elements should be carefully regulated. To produce fine-grained Mg alloys with a large size scale, new continuous severe plastic deformation (SPD) techniques must be developed. The Mg breakdown rate could be controlled by the development of a multifunctional coating. Further, the upcoming research challenges and trends for magnesium biomaterials were suggested. The shortcomings of the numerous strategies to improve the corrosion property of biological magnesium and its alloys were also evaluated.
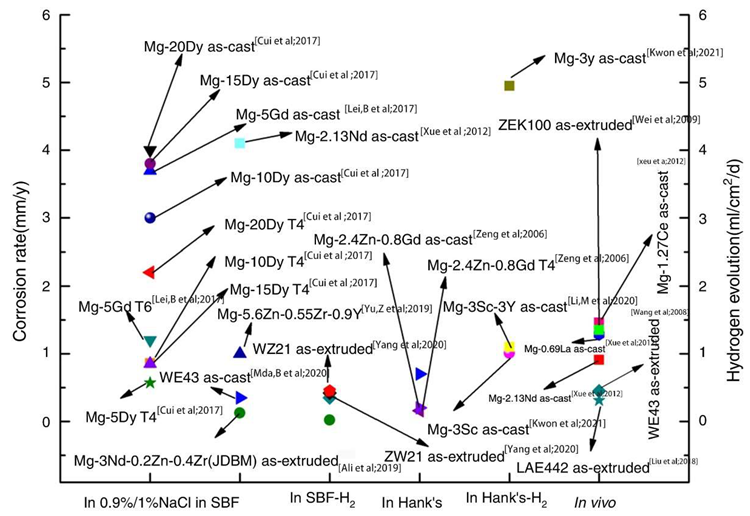
Corrosion rates of selected Mg-RE alloys in 0.9%/1% NaCl, in SBF, in Hank’s. Image Credit: Wang, L et al., Materials
Observations
The LAE442 and WE43 alloys that were extruded showed the least amount of in vivo corrosion. When the volume of LAE442 was decreased by 15.2% over the course of the three-month in vivo experiment, its strength was reduced by 40%. When the Gd addition concentration was 1.6%, the ZK60 alloy's corrosion resistance was at its lowest.
Ca had a lower standard electrode potential than magnesium at -2.76 V. The alloy's corrosion potential was reduced, and the corrosion rate dropped to 11.8% when the Ca content reached 6%. When 0.5 wt% Mn was added, the hydrogen evolution rate during the first four days of immersion was much lower than it was for the Mg-5Al alloy. When the total Zn content was higher than 2.5%, it had a negative impact on the corrosion behavior of the Mg alloy.
The Mg alloy's cathodic hydrogen evolution reaction was suppressed when its composition was less than 1 wt%. However, when the Zn content was increased to 5%, the alloy phase was created, and the rate of hydrogen evolution was increased in the cathode area. It was discovered that when the casting temperature was between 630 and 680 °C, the concentration of Fe could be greatly lowered.
A purified alloy was less likely to include impurity elements, which lowered the risk of micro-galvanic corrosion and increased the matrix's corrosion resistance. Zn, Mn, Re, Ca, and other alloying elements could effectively prevent the precipitation of grain boundaries in the second phase and raise the matrix's electrode potential by being added to the Mg alloy. The density of the oxide coating on the surface also increased due to the concentration of alloying elements on the surface, which improved the matrix's protection. However, it was important to carefully monitor the concentration of micro-alloying components.
Superfluous substances were dangerous, particularly when utilized as stents when implanting them in blood vessels. It was important to define the vital value of each piece in further detail. Extrusion, rolling, SPD, and other deformation processes could refine the grain of the alloy. Partial or complete recrystallization occurred in the hot working process of the alloy. Local corrosion was less likely because the second phase's dispersion in the matrix was fine and homogenous. However, the majority of SPD technologies could only create tiny samples.
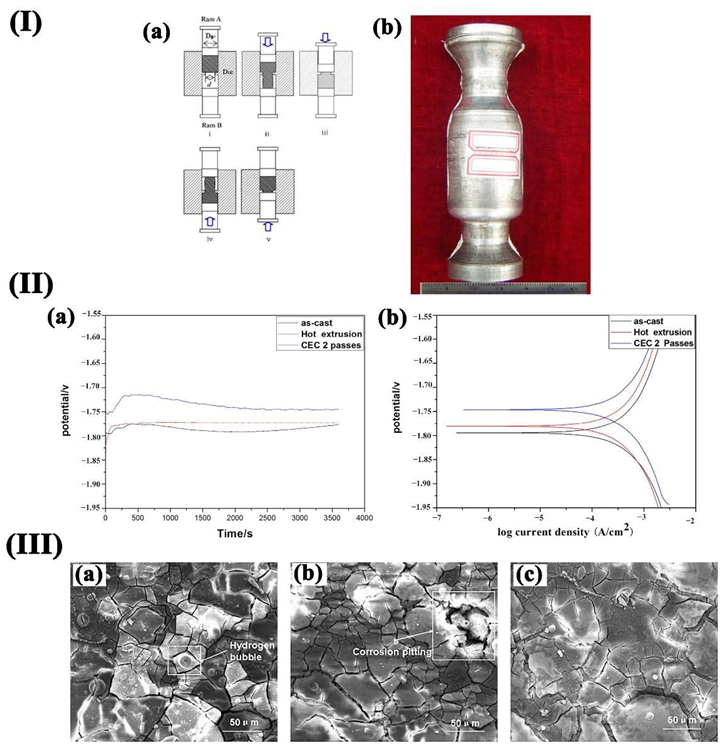
(I) (a) Schematic illustration of the CEC procedure; (b) 2 passes CEC specimen; (II) OCP (a) and Tafel (b)curves of various samples in SBF solution at 37 °C: (III) SEM images of samples after immersion for 48 h: (a) as-cast, (b) hot extruded, (c) CEC 2 passes. Image Credit: Wang, L et al., Materials
Conclusions
In conclusion, this study analyzed the properties and state of development of biomedical Mg alloys. The varieties of Mg and its alloys and the corrosion mechanism were outlined. Mg alloys frequently experienced corrosion such as pitting, intergranular, and galvanic corrosion. However, the corrosion causes were still poorly understood, particularly while it was in use, and this needs more research.
The authors mentioned that the current coating on the surface of the magnesium alloy only served one purpose and was unable to simultaneously satisfy the needs of many materials. They stated that future research will therefore concentrate on creating multifunctional coating preparation techniques with high levels of hardness and corrosion resistance.
The team advised that further research into corrosion mechanisms be done as currently no purification technique that could eliminate all contaminants exists.
References
Wang, L., He, J., Yu, J., et al. Review: Degradable Magnesium Corrosion Control for Implant Applications. Materials, 15(18), 6197 (2022). https://www.mdpi.com/1996-1944/15/18/6197
Disclaimer: The views expressed here are those of the author expressed in their private capacity and do not necessarily represent the views of AZoM.com Limited T/A AZoNetwork the owner and operator of this website. This disclaimer forms part of the Terms and conditions of use of this website.