Sponsored by HORIBAOct 18 2013
Pure carbon can exist in several forms, which are being explored for use in newly engineered applications. Raman spectra are useful to study these carbon forms even in trace quantities or in thin films. This article discusses the application of Raman spectroscopy for carbon characterization in the design, monitoring, and control of production processes.
Diamond
Diamond, created by an infinite extension of sp3 carbon-carbon bonds, is the natural hardest material exhibiting high strength, corrosion resistance, high thermal conductivity, and optical transparency. It becomes a semiconductor when doped with boron, nitrogen or phosphorous, and demonstrates electrical insulation properties in its pure form. These properties make it interesting for use in engineered materials. Nevertheless, the requirement for high temperatures and pressures for growth makes the material economically not feasible for most applications.
There are demonstrations of growing diamond films in CVD reactors under close-to-ambient conditions. Conversely, the diamond deposition is often accompanied with much sp2-bonded carbon because the material does not have a thermodynamically stable phase. Since, Raman spectroscopy is known to be more sensitive even to trace amount of sp2 carbon, it is the technology of choice to probe these films. The Raman band of the diamond also provides more information. In a single crystal diamond, the Raman line is observed at 1332cm-1. Users of Raman spectroscopy can create a quality index to measure the following characteristics by characterizing these films:
- Diamond frequency
- Diamond linewidth
- Sp2 carbon background
A characteristic Raman spectrum of a diamond film composed of non-diamond carbon in significant quantities is illustrated in Figure 1. The spectrum has been band-fit to measure the contributions of different components.
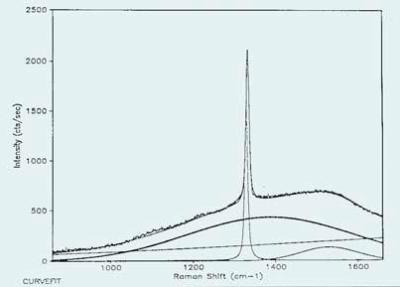
Figure 1. A typical Raman spectrum of a diamond film composed of non-diamond carbon in significant quantities.
Graphite
Graphite is created from stacks of infinitely extended planes of fused aromatic rings. The weak Van der Waals bonding between the planes causes the planes to lip, making the material to be used as a solid lubricant or as an additive to an oily lubricant. The graphite first order phonon at around 1580cm-1 is observed in typical Raman spectra carbon allotropes shown in Figure 2. This line has been recorded to move with temperature and stress. Moreover, it is difficult to decouple stress- and temperature- induced effects due to the possibility of heating by laser absorption.
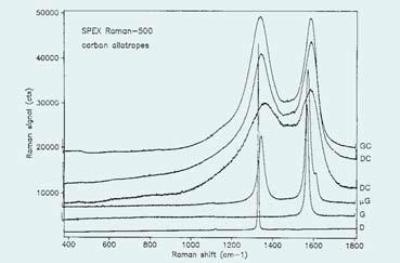
Figure 2. Typical Raman Spectra of Carbon Allotropes
The disruption of the long range order of the graphite lattice results in a second line at about 1360cm-1. There is a great variation in the relative peak intensities of lines at 1580 and 1360cm-1, referred respectively as G and D lines in the literature (graphite and defect). Additionally, there is a shift in the D band with excitation wavelength, appearing at lower frequencies with longer wavelength excitation lasers.
There is also a variation in the linewidths of these lines as the relative intensity is identified by degree of long-range order of the lattice. As a result, it has in recent years, been considered more appropriate to measure the ratio of the integrated intensities of the lines utilizing bandfitting algorithms to decouple the components. Carbons exhibiting this behavior is called disordered carbon (DC). Figure 2 shows Spectra of two samples.
Furthermore, it has been demonstrated that some carbon films need a second defect band at around 1500cm- 1 to flatten the residual of the band-fitted Raman spectra. If band-broadening and overlapping in carbon films is widespread so that separate bands are not visible prior to the band-fit, then the material is termed as ‘diamond-like carbon (DLC).’ DLC films have found use as passivation layers in magnetic storage devices.
Glassy Carbon (GC) is a material having the intensity ratio of the D and G bands reversed (i.e., D band is higher). With fairly sharp bands, this material contains highly crystalline regions, yet with very small dimensions. A spectrum of glassy carbon is also illustrated in Figure 2. Since the D and G bands are related to various sizes of graphitic structural units, improvement of the two Raman bands that relies on the proximity of the laser wavelength to electronic transitions is likely to show a different behavior when there is a change in the excitation wavelength.
Engineered Carbons
It is possible to use the Raman spectra to measure the degree of structural order and orientation in technologically significant carbons. Spectra of carbon fibers produced from pitch and PAN (polyacrylonitrile) illustrated not only a disparity in long range order but also polarization-dependent intensities associated with the orientation of the graphitic planes in the fibers.
Hard carbon films are widely used in the production of hard discs and on read-write heads. Engineering the deposition equipment parameters is controlling the tribological and physical properties of the films. These properties can be rapidly confirmed with the help of Raman spectra because of the correlation of the spectra with the tribological properties of interest. Figures 3a and 3b show carbon film spectra that need a fit with two or three bands, respectively.
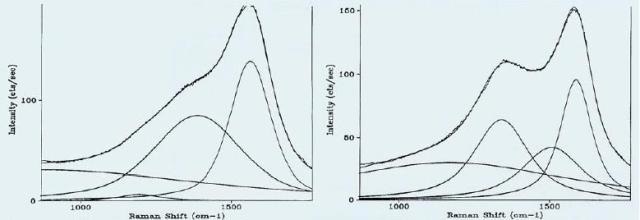
Figure 3. Raman Spectra of Carbon Films
Buckeyballs or Fullerenes, Nanotubes
It is possible to build capsules, tubes and balls of graphitic-like fused aromatic rings. The Raman spectra of some of these substances have been acquired from films of pure materials. The Raman spectra of these materials consist of many fairly sharp bands In contrast to the Raman spectra of other carbon forms, which comprise at most three bands in the fingerprint region of the spectrum (100- 1800cm-1).
The spectra of carbon nanotubes reveal the material’s structure, which decides their semiconducting and metallic properties. These materials show promise in applications, including nanoelectronics and in several functional devices such as field emitters.
Conclusion
The high sensitivity of the Raman spectra of the different forms of elemental carbon to the type of nearest neighbor bonding, and to intermediate and long range order makes Raman spectroscopy the technology of choice for carbon material characterization in many cases. The correlation between Raman spectral features and tribological properties can assist the deposition of carbon films.

This information has been sourced, reviewed and adapted from materials provided by HORIBA.
For more information on this source, please visit HORIBA.