In cylinder gases, low ppb gas detection limits for contamination have been accomplished with gas chromatography using a modified gas injection system as well as megabore capillary columns with a range of detectors such as atomic emissions, mass spectrometry, and discharge ionization. The required components are separated in a single run through a special valve switching scheme.
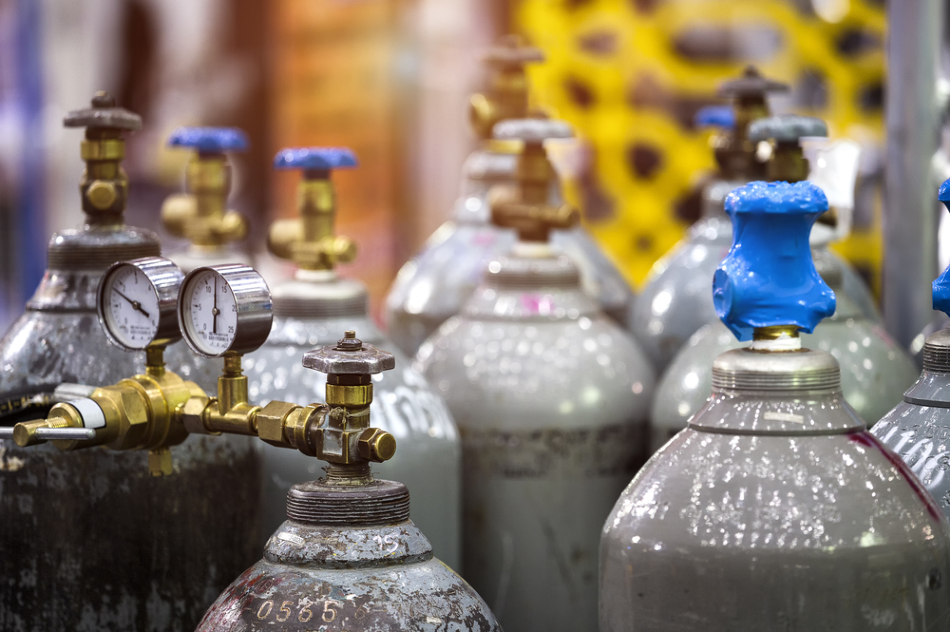
Image Credit: Shutterstock/tool2530
A gas blending unit, controlled by computer, offers several levels of dilution of standard gases, enabling the generation of complete calibration curves with excellent accuracy and precision. Using the methods and equipment described here, reproducible detection limits below 50 ppbv are typically accomplished.
In the semiconductor manufacturing industry, the increasing demand for large-scale integration has enforced the need for chemical purity, driving the technology for higher sensitivity in the field of gas analysis. Laboratories that perform analysis on cylinder gases have been traditionally using dedicated analyzers for their work, but have now switched to gas chromatography (GC) that comes with a wide range of detectors. Ionization detectors including flame (FID), thermal conductivity detectors (TCD), helium (HID), discharge (DID), and photo (PID), together with ultrasonic detectors (USD) have been used to characterize gas samples. Most laboratories tasked with these types of analyses have switched to GC with one or more of the detectors described above. While instruments such as electrolytic oxygen analyzers, reduction gas analyzers, and similar dedicated analyzers provide remarkable sensitivity, they do not provide much information about the gas under scrutiny. Moreover, it will be relatively expensive to purchase these types of dedicated analyzers to get a broader view.
Performing full-scale analysis, such as that afforded by chromatography, can provide a better understanding about the contamination present in the gases of interest. Almost all chromatographs are designed for the analysis of liquid samples, but this design characteristic poses problems for gas analysts. This is because instrumental sensitivities are usually below than that needed for trace-level analytical work on gaseous samples, and the hardware also has to be modified before introducing the gas phase samples into the instrument.
This article describes a chromatographic method and instrument modifications that have resulted in the development of capabilities for routine analytical testing of cylinder gases at trace level.
Experiment
Chromatography
A Hewlett Packard 5988A GC/MS was employed throughout this study.
The 5890 GC’s stock sample inlet was substituted with a Valco ten-port gas injection valve with a 250 ml sample loop. The valving scheme utilized in all of this work is shown here in Figure 1. This arrangement enables baseline separation of key components of air in one 16-minute run. Separations are achieved using two columns, in series. Chrompak PoraPLOT Q is the first column, followed by a Molsieve 5A column from the same manufacturer. These are 0.53 mm OD x 0.33 mm ID x 25 m fused silica "Megabore" capillary columns.
Mass Spectrometry
A third party system from Teknivent replaced the stock data system and software of the 5988A because the accuracy and reproducibility of the Teknivent Vector/2 software integration routines were shown to be more reproducible and accurate at the levels of interest in this work. Electron energy is employed as a tuning variable because the acquired spectra are not subjected to library searches or used for comparison. An electron energy of 58 eV was employed for most part of this work and this value is much greater than the ionization potential of all species of interest in these analyses. For tuning/calibration purposes, the m/z 18, 28, 32, and 44 peaks of air were utilized along with perfluorotributlyamine (PFTBA) as the contaminants of interest are below m/z 69. For this operation, adequate air is provided by normal system leakage. A calibration report is shown in Figure 1, listing all parameters for a typical tune file employed in routine analyses conducted in this laboratory. Also shown are three of the peaks employed for tuning and calibration purposes, such as nitrogen (m/z = 28), water (m/z = 18), and carbon dioxide (m/z = 44).
Results and Discussion
Air components are the major contaminants involved in most areas of gas analysis. Oxygen, nitrogen, carbon dioxide, methane, carbon monoxide, and many others at lower concentrations are given more importance when investigating cylinder gases to the sub- ppm level. Apparently, the process of filling a cylinder with a product gas while surrounded by a "sea" of air is not easy. The same issues were faced when attempt was made to recover a representative sample from a cylinder and deliver it to an instrument for further analysis. To ensure that sample is not contaminated with air - the omni-present contaminant - system mechanical integrity should be of the highest level. Toward this end, dreary schemes for minimizing system-sampling leakage have been developed. Here, the term "minimize" is used to highlight the fact that system leakage cannot be avoided completely. All systems will leak at some level and hence, it will not be possible to create a truly "leak-free" system when conducting trace-level gas analysis.
Only a few analytical instruments are available on the market and these are provided by the manufacturer in the appropriate configuration for conducting gas analysis at the trace levels. Therefore, almost all equipment used for this purpose had to be modified before utilization. While most of the required modifications are carried out on the inlet system, some other variations from convention are used in this work. When the 5890 GC arrives from the factory, it is assembled for liquid injection. First, the liquid injector has to be removed and substituted with an appropriate gas injection system. The design of the chromatographic separation is a key consideration in this modification. The chromatography used for separating the components of air from an inert balance gas such as nitrogen or argon does not pose much difficulty.
In this analysis, the PoraPLOT Q (PLOT) column is used to separate carbon monoxide and methane from other prime components of air.
The eluents from a sample injected onto a PLOT column include one peak for oxygen, neon, argon, nitrogen, and carbon monoxide (Figure 2). This "air" peak is followed by methane, and subsequently by carbon monoxide. Further separation of the "air" peak is done by the Molsieve column and carbon dioxide is irreversibly retained. Hence, the separation should be arranged in such a way that carbon dioxide is not exposed to the Molsieve column. The injection valve arrangement, depicted in Figure 3, can be used to achieve this separation. Leveraging this configuration, the sample is introduced onto the PLOT column as the valve is changed from the Load position to the Inject position. This executes the initial separation illustrated above. The valve is returned to the Load position before the carbon dioxide enters the Molsieve column and the methane clears the PLOT column. A pretest injection is performed on an "as necessary" basis to determine the timing of this event, typically soon after calibration or tuning as shown in Figure 4. The valve switch forces the carbon dioxide gas to be "short circuited" directly into the mass spectrometer, preventing the Molsieve column altogether; the remainder of the constituents are directed toward the Molsieve column, where more separation occurs. It must be remembered that the direction of flow via the columns is never reversed, as doing so can degrade chromatographic resolution. The separated components, after eluting from the Molsieve column, again pass via the PLOT column. Without flow-reversal, this additional trip cannot be prevented but it does not cause any adverse effects. The separation of a 100 ppb standard multicomponent blend, utilizing the method mentioned above, is shown in Figure 5. Normally, detection limits below 50 ppb are achieved.
Similar to all trace level gas analysis, system leakage is very important to achieve adequately low background levels to facilitated low detection limits. Toward this end, a number of measures have been adopted which minimize system leakage, or at the most, attenuate the effects of such leakage. For this type of work, it would not be wrong to assume that there is a truly "leak free" system. At the limit of reality, almost everything leaks, to some extent. Although some types of fittings can seal better than others, all fittings are believed to be sources of leakage. Based on this fact, and given that all connections cannot be fully eliminated from this type of system, all fittings in the chromatographic stream are placed in a purged housing over the chromatograph kept under pressure with a few psi of ultrapure helium in a customised, clear plastic housing. Some fittings in the chromatograph flow stream which are not placed in this housing include those at the purifier for incoming carrier gas and the connection at the interface between the mass spectrometer and the GC. Apart from this measure, the number of fittings has been further reduced by joining the GS/MS transfer line and all chromatographic columns directly into the ports of the injection valve, without having to adapt from capillary column to l/16" stainless steel tubing. This made it possible to remove eight fittings from the flow stream. This interconnection was facilitated with the use of glass-filled Teflon ferrules from Scientific Instrument Service. The (green) ferrules enable this direct connection more readily than ferrules fabricated from other materials. These interconnections should be made carefully, since there is always a possibility that the end of the capillary column will be pushed too far into the valve head, which can crush the end of the column or cause a scoring to the valve rotor. During the column installation process, the removal of preload assembly and rotor enables the operator to make sure that the column is properly placed in the valve assembly and this can be done by looking down into the rotor seating area during and observing the placement of column.
The interface between the mass spectrometer and the GC is also unique, in that there is no form of separator or other eluent splitting involved. The transfer line includes 10 m of 0.2 mm I.D. fused silica capillary column linked to a port on the injection valve and directly routed into the mass spectrometer. All eluent from the chromatograph is directed toward the mass spectrometer, producing base pressures that are usually on the order of 2 x 10-6 torr.
Calibration is achieved with the help of a microprocessor-based Environics Gas Blender. This specific unit is the culmination of several years of association with Environics, Inc. (Tolland, CT). The original commercial unit contained standard-grade stainless steel tubing, Swagelok connections, industrial-grade solenoid valves, and mass flow controllers with polymeric seals. However, these construction materials were not suitable for calibration of gas generation in the area of interest for this work. Refinements include replacement of all unavoidable fittings with metal-seal VCR connectors and a fitting minimization scheme. The entire tubing of the system is chemically cleaned and electropolished, and joints are orbitally welded. In addition, the system includes mass flow controllers that are made of all-metal seal construction, and features solenoid valves that are zero dead volume bakeable T-solenoids. The unit is designed in such a way that each individual gas circuit is continuously and automatically purged with high purity helium when it is not exactly involved in the dilution scheme. This feature reduces memory effects, enabling the user to achieve the preferred concentrations more quickly. These refinements have considerably enhanced the unit’s ability to yield reliable dilutions of a multicomponent calibration gas over many orders of magnitude with excellent reproducibility and accuracy. In applications like these, a calibration cylinder containing 50 ppm each of 8 components in a balance of helium are dynamically diluted in five steps over a range of 1 ppm to 50 ppb to create calibration curves spanning the area of interest. Additionally, calibration acceptance is based on acquiring a correlation coefficient for a linear least-squares-fit line through the six data points (forcing zero) of at least 0.995. This is followed by introducing the analytical sample into the injection valve sample loop via the same pathway as the calibration standard, through the gas blender, so as to guarantee consistency of sampling.
Conclusion
This work has shown how routine quality control testing of cylinder-based gas phase products can be performed with the required accuracy, precision, and flexibility essential for screening a wide range of gases employed by the semiconductor sector. The purity specifications for gases investigated in this laboratory range from several ppm to below 100 ppb for the contaminants given above. The chromatographic methods and modified instrumentation described in this article make it possible to conduct routine QC screening for different grades of helium, nitrogen, and argon cylinder gases. The advanced gas blending system considerably contributed to the success of this work. For this type of analysis, dependence on common "zero-and-span", two-point calibrations has been shown to be undesirable at these levels. Further plans are to apply these methods for analyzing non-inert gases, including corrosive, pyrophoric, and/or toxic gases.
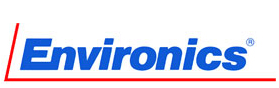
This information has been sourced, reviewed and adapted from materials provided by Environics, Inc.
For more information on this source, please visit Environics, Inc.