.jpg)
Image Credit: SvedOliver/Shutterstock.com
Thin films of oxide and organic materials are the building blocks of a wide range of nanomaterials and devices in battery technology, sensors, and optoelectronics.
Currently, there is a huge shift towards research concerning the development of thin films based on oxide, organic (polymers or small molecules) and metallic thin films. Thin-film materials measuring a few nanometers or a single molecule worth of thickness can display exciting electronic properties enabling the development of novel technology. Typically, these properties are related to the unusual arrangement of the polymer backbones, and ultimately to the optical transition dipoles inside the nanostructure of the thin film.
One of the largest areas of growth for organic thin films is optoelectronics, which includes inorganic semiconductors, such as III-V or silicon compounds, and also ferroelectric oxides and low-cost ‘plastic’ organic semiconductors. Typical applications in this key area include sensors, photoresistors, photodiodes and solar cells (energy harvesting), phototransistors, charge-coupled imaging devices, new types of lasers and low energy lighting.
Determining the Properties of Thin Films
The deposited thin films of various materials have to be characterized and analyzed in order to determine their appropriateness for purpose and the extent of their chemical and electronic properties. Surface vibrational spectroscopy is considered as one of the most powerful tools for acquiring direct data on the structure of the adsorbed molecule on solid surfaces and their overt bonding character.
Infra-red reflection absorption FTIR spectroscopy (IRRAS) can be handled in relatively high-pressure situations and has become an important tool for carrying out real surface studies. Polarization modulation (PM) methods have been used to improve the sensitivity of IRRAS. This means PM-IRRAS FTIR is essential for high-sensitivity detection of adsorbates on metal surfaces in absorbing gaseous media.
This method makes it possible to observe weakly surface adsorbed species and metastable reaction intermediates, which continue when gaseous molecules are present at room and high temperatures. This enables the determination of the film’s chemical characteristics and also gives an indication of the formation reactions. PM-IRRAS FTIR is an outstanding technique for the analysis of nanometer-scale thin films. With this technique, users can obtain FTIR spectrums of films of approximately 2 nm thick and deduce molecular orientation.
How Polarization Modulation Enhances FT-IR
The polarization modulation‐infrared reflection‐adsorption spectroscopy (PM‐IRRAS) technique can be employed to acquire the reflectance spectra of thin polymer films and spontaneously arranged monolayers adsorbed onto metals such as chromium, silver, and gold.
Differential PM-FTIR reflectance spectra are acquired by photo-elastic modulation of the FTIR beam polarization and a real-time sampling methodology in order to produce the average and differential FTIR interferograms from Infrared signal measurements during each modulation cycle. Using polarization-independent optics after the metal surface, users can obtain a true surface Infrared differential reflectance spectrum. This method has the benefit of high surface sensitivity, as well as of the surface selection rule, where a number of infrared absorption bands are missing from the spectra of molecules adsorbed on metal particles.
Furthermore, polarization modulation removes background signals from environmental factors such as CO2 and water vapor. Therefore, there is no requirement for a protective gas atmosphere or the optical part of the FTIR spectrometer to be in a vacuum. Since changes in water surface height or changes in the light source intensities do not disturb the final spectrum, longer measurement times are also possible.
Why Must the Light be Polarized?
The IRRAS technique is dependent upon the angle of incidence, the optical constants of the substrate and thin film, and the polarization of the incident IR radiation. A photo-elastic modulator produces alternating linear states of polarized light, the so-called s and p components of radiation where s refers to perpendicular polarized radiation and p to parallel polarized radiation.
A typical setup comprises of two-wire grid linear polarizers (for instance, polarizing filters from Specac) creating a crossed analyzer setup, a PEM further modulating the polarization state, and the optical sample introducing the modification in the polarization of light. The final detected intensities at the second and fundamental harmonic of the PEM operating frequency rely on the rotation and ellipticity introduced by the sample.
The phase change of the perpendicular component, s, shows no major dependence upon the variation of the incidence angle, because the phase change of the perpendicular component, s, is almost 180° for all the incidence angles, and the net amplitude of the IR radiation parallel to the surface of the substrate is zero. On the contrary, the phase change of the parallel component, p, strongly depends on the incidence angle.
The p-polarized component experience a maximum at 88° resulting in a net combined amplitude that is nearly twice that of the incident radiation. This feature provides the improved differential reflectance spectrum of the adsorbed surface species, that is, the thin film.
Filters for Polarization Modulation
PM-IRRAS FT-IR experiments need polarization filters where the transmittance of the filter stays constant despite the polarization state of the infrared radiation, and optical throughput is also high. Specac polarizing filters allow a high resolving power, which enables discrimination of vibrational bands very near, particularly at short wavelengths.
.jpg)
Infrared Polarizers from Specac
Specac IR grid polarizers are best because they provide high transmission efficiency, a high degree of polarization: > 99.9%, a broad wavelength range (20 µm to 10 mm), superior resolution, and no beam dispersion or deviation.
Download the Brochure for More Information
References and Further Reading
- T. Buffeteau, B. Desbat, and J. M. Turlet, "Polarization Modulation FT-IR Spectroscopy of Surfaces and Ultra-thin Films: Experimental Procedure and Quantitative Analysis," Appl. Spectrosc. 45, 380-389 (1991)
- Barbara J. Barner, Michael J. Green, et al., Polarization Modulation Fourier Transform Infrared Reflectance Measurements of Thin Films and Monolayers at Metal Surfaces Utilizing Real-Time Sampling Electronics, Anal. Chem. 1991, 63, 55-60 55
- Aritada Hatta, Toshimasa Wadayama, et al., A Polarization Modulation Infrared Reflection Applied to Study of Thin Films on Metal and Semiconductor Surfaces, Analytical Sciences December 1985, Vol. 1, 403
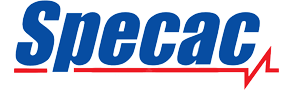
This information has been sourced, reviewed and adapted from materials provided by Specac.
For more information on this source, please visit Specac.