Sponsored by HORIBAJun 14 2018
Raman microscopy is an analytic technique which uses a standard optical microscope in combination with a Raman spectrometer to visualize samples under high magnification as well as performing Raman analysis of the specimen using a laser spot of microscopic size. The method itself consists simply of placing the sample beneath the microscope objective, focusing on it and measuring as required.
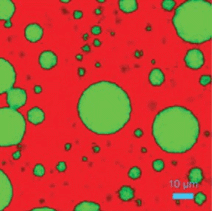
Cross section analysis of commercial plastic, showing the capability of confocal Raman microscopy for analysis of µm thick layers
However, this setup doesn’t allow for control over the volume sampled. This can be achieved by adding a spatial filter in what is called confocal Raman microscopy. This achieves spatial filtering of the volume of the sample analyzed, in both XY and Z axes (referring to the lateral and depth axes respectively).
Many methods are used currently to apply this technology, including true confocal aperture, or pseudo confocal slit-binning. While some may be claimed to be superior to others, it is well known that true confocal Raman microscopy can allow individual particles or layers as small as 1 micron or less to be analyzed.
With true confocal Raman microscopy, the spatial resolution varies with the laser wavelength and the quality of the laser beam in use, as well as other parameters such as the type of microscope objective selected. When the high-magnification objective is properly matched to the visible exciting laser, optimal settings allow the highest possible spatial resolution to be achieved, typically within 0.5 to 1 micron.
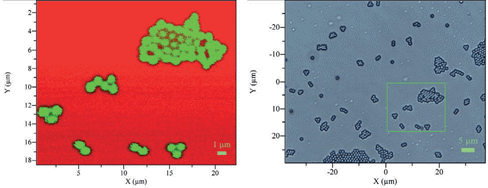
A confocal Raman spectral image of 0.9 µm polystyrene beads
Remote in-situ Raman analysis
Remote in-situ Raman analysis refers to the technique of direct in-situ or remote analysis of a sample without having to take an extract of the sample to a Raman spectrometer. This is ideal for industrial use where components undergoing reaction in a reaction vessel can be monitored, whether in a small glass flask or a reactor built on industrial process lines. Or chemicals could be analyzed at several points within the pipelines transporting them.
This analysis may be and is often performed using optical fibers. This allows the connection of a remote spectrometer to a compact but durable Raman probe head at the sample site which could be several hundred meters away. The laser is carried to the sample via a single cable, while a second fiber carries the Raman spectra to a standard spectrometer and detector system. This pair of cables is joined to the Raman probe head. Thus this system carries the laser signal to the sample, focuses it, and collects it.
These probes are built to withstand heat and pressure, and may be used in immersion mode with the head placed inside the reaction fluid system, or as a stand-off system where the laser is focused through a clear window placed in the reaction vessel or in the pipeline where the sample is found.
Some applications of in-situ Raman analysis include:
- Emulsions
- Headspace analysis within reactors or vials
- Mixtures of reagents or solutions
- Suspensions and slurries
- Material feeds, raw or bulk
- Effluents from vessel cleaning
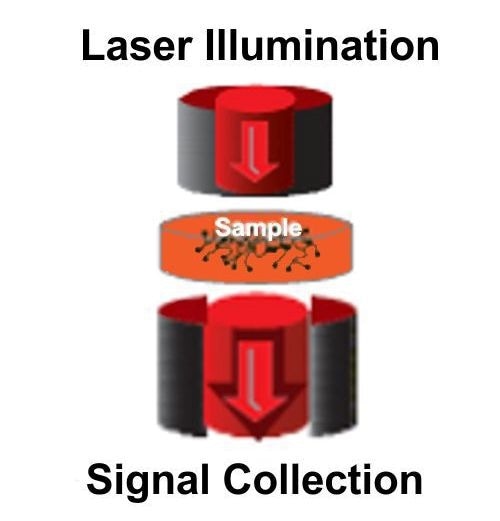
TRS schematic
Transmission Raman Spectroscopy
Transmission Raman spectroscopy, or TRS, refers to another form of Raman analysis which helps to analyze bulk materials which are turbid or opaque. It is based on the principle of collecting the Raman light waves moving through the sample material in the same direction as the exciting laser which illuminates it, on the side opposite to the entry of the laser beam.
The opacity of the sample does not hinder the passage of the laser beam, which is scattered as it propagates. Many of the scattered photons undergo the Raman effect and this data is used for TRS.
The geometry of such transmission allows the true bulk to be measured using the whole sample volume, as from, for example, a tablet of a drug, which makes it superior to conventional Raman spectrometry-microscopy systems.
Other advantages of TRS include its non-invasive and non-contact nature, which leaves the sample intact. It is also independent of sample preparation. Additionally, it remains unchanged by the effects due to particle size, the homogeneity of the sample, and the orientation.
Some common uses of TRS include:
- API concentration
- Determining the uniformity of sample content
- Polymorphism
- Crystalline nature of the sample
- Composition and purity of a powder
- Transmission Raman spectroscopy can be used to understand:
- Solid form
Resonance Raman Spectroscopy
Resonance Raman spectroscopy is a modified form of Raman spectroscopy which uses any wavelength of laser to excite the sample and measures the Raman effect on the emerging light. The result of such analysis remains relatively unchanged despite the use of a range of wavelengths, but the variety of wavelengths does create some problems with the technology.
This is countered by resonance Raman spectroscopy in which the exciting laser has a selected wavelength that is so close as to almost or completely overlap an electronic transition. This is typically found to be in the UV-visible region. Such an overlap leads to intensities of scattering which are higher by an order of 102-106 compared to normal Raman analysis. This helps expand the limits of detection and cut down measurement times.
The difficulty is that this also contributes to more significant background fluorescence which creates more problems than with the conventional method.
Another method that achieves similar increases in signal intensity is Surface Enhanced Raman Scattering (SERS), which has the advantage of less fluorescence in the background.
Resonance Raman spectroscopy is ideal for some applications such as detection of environmental pollutants in concentrations as low as ppb or ppm.
Any standard Raman spectroscopy system can be adapted to resonance Raman and the standard method of measurement is used, but with the use of suitable excitation laser wavelengths.
Tip Enhanced Raman Scattering, or TERS
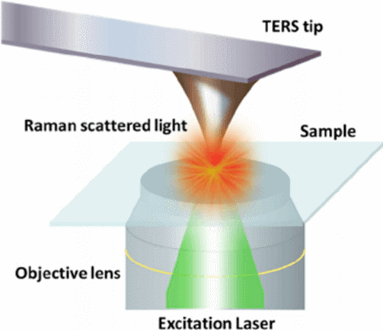
Reflection and Transmission TERS configurations
TERS (Tip Enhanced Raman Spectroscopy) is a technique which allows imaging resolution at nanoscale using Raman spectroscopy. It also avoids the necessity of using a label to achieve excellent resolution, making it capable of becoming a top contender among emerging imaging technologies.
TERS uses an AFM/Raman system that integrates a Scanning Probe microscope (SPM) with a confocal Raman spectrometer using optomechanical connections. An SPM is capable of being used in different modes, namely atomic force, normal or shear force mode, or scanning tunneling mode, and it provides ultra-high resolution.
The optical coupling is responsible for the arrival of the exciting laser beam to the probe tip, while the spectrometer analyzes the light that is scattered, giving a hyperspectral image with chemical contrast at nanoscale.
The foundation of TERS is a tip typically made of gold or silver (though other metals are sometimes used) which brings the incident light field to a focus at the probe apex. This therefore behaves as a nanoscale source of light as well as enhancing the local field.
This increases the sensitivity of the Raman field by an order of 103–107 while confining the volume probed by the tip to a nanoscale region located just below it. The optical coupling between the SPM and the spectrometer achieves confocal behavior by one of two possible configurations: transmission and reflection (as shown in Figure 28). Each of these has its own advantages and limitations.
The use of the transmission configuration means that the objective used, including the immersion objective, can have the highest numerical aperture (NA). The focus will then have a high power density so that a high signal level can be collected. However, this is suitable only for transparent samples. The reflection configuration is not limited in this way, both opaque and transparent samples being analyzed, but the objectives used must have lower NA.
When scanning from point to point is combined with spectrum acquisition at the same time, Raman mappings can be performed in the near field while achieving lateral resolution to 10 nm or less.

This information has been sourced, reviewed and adapted from materials provided by HORIBA.
For more information on this source, please visit HORIBA.