Micellar water is the fashionable new facial cleanser boasting an ability to clean skin without causing irritation. This article introduces results from dynamic light scattering (DLS), phase analysis light scattering (PALS), and microrheology quantifications of commercial micellar water formulations utilizing the Brookhaven Instruments NanoBrook Omni system.
The charge and size of the micelles might affect their stability and performance. This technology could offer a novel means of easily characterizing these formulations.
Micellar water is currently a fashionable facial cleanser on the cosmetic market. Numerous companies sell this commodity, touting its capability of removing dirt, oil, and makeup without leading to skin irritation. As demonstrated in Figure 1, micellar water is administered to a cotton pad and subsequently applied across facial skin, removing impurities in the process. The primary constituents of this commercial cleanser are water, surfactant, and moisturizer, with fragrance regularly included.
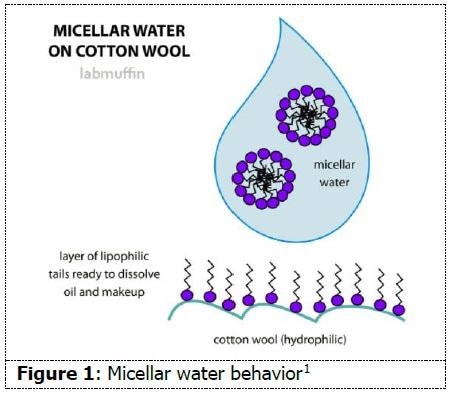
Micelles form when an aqueous solution possesses a particular quantity of surfactant molecules. The hydrophilic heads of the surfactant molecules align themselves towards the water molecules, whereas the hydrophobic tails align towards each other, thus circumventing water contact. These spherical aggregates are broadly utilized in cosmetics, water treatment, drug delivery, detergents, and more.
Characterizing these micelles can thus assist in qualifying their performance. A regular method of characterization for solutions such as these is the use of non-invasive laser light scattering. This method can be utilized to quantify particle charge, size, and rheological properties.
At present, it is unclear whether a qualification or characterization methodology of micellar water formulations has been determined. The occurrence of micelles and the diluted nature of the solution suggest it could be characterized by laser light scattering. In this article four commercial micellar water formulations and one control formulation were quantified by DLS, PALS, and microrheology, with representative results displayed herein.
Material Methodology
Four commercial formulations of micellar water were acquired from a local vendor (Samples 1-4). One formulation was prepared to comprise the three primary ingredients of micellar water to operate as a control (Sample 5).2 Each of the five formulations were poured directly into ordinary cuvettes for quantification.
Analysis was undertaken with a NanoBrook Omni utilizing dynamic light scattering (DLS) for particle size analysis, phase analysis light scattering (PALS) for zeta potential analysis, and microrheology for viscoelastic analysis, with the Particle Solutions software suite. Three DLS quantifications, five PALS quantifications, and three microrheology quantifications of each sample were generated to guarantee reliability and repeatability of results.
Results
DLS
A baseline was determined with initial measurements at the 90° angle and 5 μs first delay time. The measurement conditions were subsequently enhanced by implementing the backscattering angle (173°) and 0.5 μs first delay time to enable full interpretation of particle sizes under 20 nm.
The correlation function is the first graph that should be examined while analyzing the integrity of a DLS measurement. The decay needs to be smooth and must flatten out to a zero slope. The occurrence of multiple decays in a correlation function establishes the presence of multiple size populations.
As shown in Figure 2, each of the correlation functions displays at least one smooth decay and flatten out. Multiple decays can be evidenced among samples 1-4. The non-negatively constrained least squares (NNLS) algorithm within Particle Solutions was administered in order to interpret the size populations present.
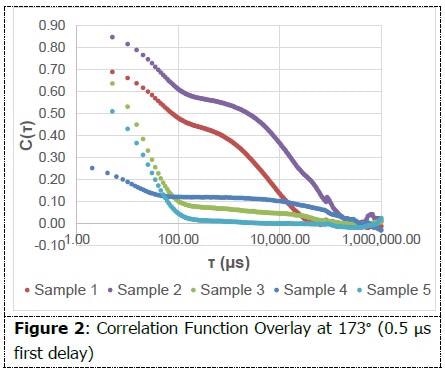
As shown in Figure 3, on an intensity-weighted basis, all samples have particles in the 10 nm range, and most also possess populations in excess of 1 micron. This data indicates the presence of small micelles in addition to aggregates or additional species. As shown in Figure 4, on a number-weighted basis, each formulation possesses a dominant 10 nm population.
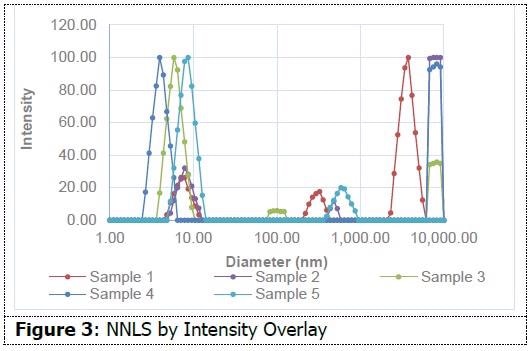
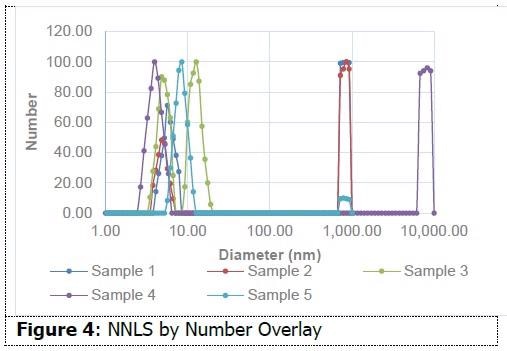
There are also fewer populations in the micron range in comparison to the intensity interpretation. This indicates that these formulations possess a high percentage of particles in the 10 nm range in comparison to those in the micron range. Sample 3 only demonstrates evidence of particles around 10 nm. This data suggests the capability of dynamic light scattering to measure size differences between micellar water formulations. This insight might prove helpful in the formulation process.
PALS
By administering a voltage to a suspension containing charged particles, measurement of the particles’ mobility is possible. This mobility value is subsequently utilized to make a zeta potential calculation. Zeta potential is determined by the charge close to the surface of the micelle. Table 1 encapsulates the zeta potential, mobility, and conductance results.
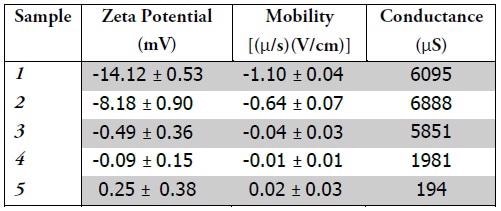
Samples 1 & 2 embody negative charge. Zeta potential magnitude can be utilized to determine suspension stability. As the magnitude of the zeta potential becomes larger, so suspension becomes more stable, as a result of the electrostatic repulsion between particles. The sign of the charge can also describe the behavior of the suspension and the particles.
Dirt is usually positively charged; the application of negatively charged micelles might enhance the efficacy of the formula. Samples 3-5 each embody neutral charge. As Sample 5 was the control, containing neither stabilizers nor additives, it is suggested that these additives would represent practical means of modifying the charge of the micelles. Either the formulators are deliberately generating a neutrally charged suspension or samples 3 & 4 might not include additives affecting the charge of the micelles.
Neutrally charged suspensions are susceptible to particle agglomeration, often causing these larger particles to settle out of solution and no longer provide effectual formulas. The conductance value is in direct relation to a solution’s ionic strength. Samples 1-3 comprise far more salt than Sample 4. Sample 5, which was the control, was not mixed with any salt, which is reflected in the measured conductance value.
Samples 1 and 2 embodied the largest charge magnitude and the highest ionic strength. These findings correspond to the fact that salt concentrations influence both charge and size of micelles, as demonstrated by zeta potential. This data suggests the capacity of zeta potential quantifications to measure dissimilarities between formulations, which might assist in the characterization of efficacy and stability.
μRhe
Microrheology is utilized to establish the viscoelastic properties of fluids. Microrheology permits the quantification of these characteristics at far higher frequencies than a conventional rheometer. As it utilizes the same principles of DLS, this experiment is straightforward.
A probe particle of an established size is selected and integrated into the solution of interest, causing it to dominate the scattering signal. The motion of this probe throughout the solution is tracked and utilized to determine rheological properties.
An 820 nm diameter latex standard (Spherotech) was integrated into each sample as the probe particle. Three quantifications were taken for each sample. Figure 5 presents an overlay of complex viscosity for one quantification of each sample. The shape of the curve for each sample suggests shear thinning, while also supporting its non-Newtonian behavior.
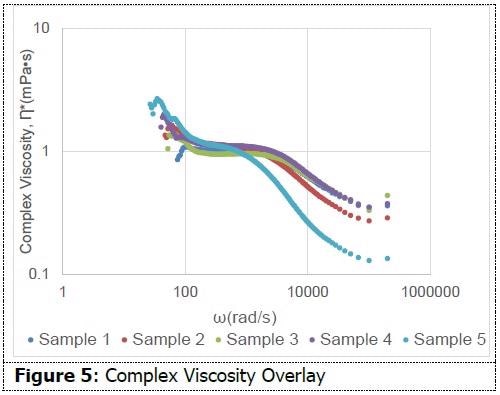
This quality might prove beneficial for micellar water since its method necessitates the use of force while wiping the face. Among all formulations, there are moderate dissimilarities in complex viscosity. This data suggests the capability of dynamic light scattering measurements to determine viscoelastic characteristics among formulations.
Conclusion
By undertaking particle size, zeta potential, and microrheological analyses, overt similarities and dissimilarities between commercial micellar water formulations were identified. The presence of 10 nm particles together with particles in the micron size range supports the presence of micelles as well as potential aggregates or additional species.
Characterization of these size populations by DLS was simple and might prove helpful in quality control and formulation to determine which populations should be present and which need to be extracted.
The presence of charge differences, identified with PALS, might prove helpful in characterizing stability and efficacy in accordance with the manufacturer’s expectations. Consistent batch-to-batch stability might also be easily qualified using this approach. The microrheological data might be utilized in conjunction with all of these methodologies for the additional qualification of formulas from batch-to-batch.
Complex viscosity and other properties might prove beneficial to the ease of application of the micellar water. Developing this preliminary work, future research can be undertaken to completely comprehend the prominent role light scattering might represent for this product and industry.
References and Further Reading
- Michelle. “What Is Micellar Water and How Does It Work?” Lab Muffin Beauty Science , 14 Sept. 2015, labmuffin.com/fact-checkwhat- is-micellar-water-and-how-does-it-work-an-update/.
- Carli, Belinda. “Creating Micellar Water.” Institute of Personal Care Science , 28 June 2017
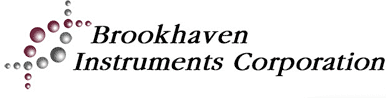
This information has been sourced, reviewed and adapted from materials provided by Brookhaven Instrument Corporation.
For more information on this source, please visit Brookhaven Instrument Corporation.