Please can you tell us about your research and studies into the heaviest elements in chemistry?
My group studies the chemistry of the heaviest elements that you can interrogate using fairly traditional chemical methods. We study the chemistry of elements like californium, berkelium, einsteinium, and fermium. These are the last elements that can be prepared in a large enough quantity that you can see with the naked eye.
What we're trying to understand is why their chemistry is different from what people thought it would be. Historically, we have extrapolated from lighter elements, what we predict the chemistry of these heavy elements will be like.
Those extrapolations sometimes work, but sometimes they fail. We're trying to understand the origin of why they fail. The information is useful in an applied sense, because if you move to slightly lighter elements - so you just slide over one element from berkelium, you're at curium and one element from that is americium and one from that is plutonium. Those elements are, of course, of significant societal interest because they're present in the used nuclear fuel that we fission in order to make electrical energy. They're also present in nuclear defence devices.
As a society we have to be concerned about their ultimate fate in the environment, and if there are things that we can do to mitigate the mistakes of the past. Every nuclear enabled nation, including the United States, England, and many others, has a legacy from the Cold War when these things were being developed. We don't have methods for dealing with all aspects of that legacy. In some cases, we have good paths forward and in other cases we don't.
At the end of the day, if you don't understand the fundamental properties of these elements, you can't treat them properly. That's really the goal of my group; to understand - at the most basic level - how these elements function in a chemical sense and also in terms of their physical properties.
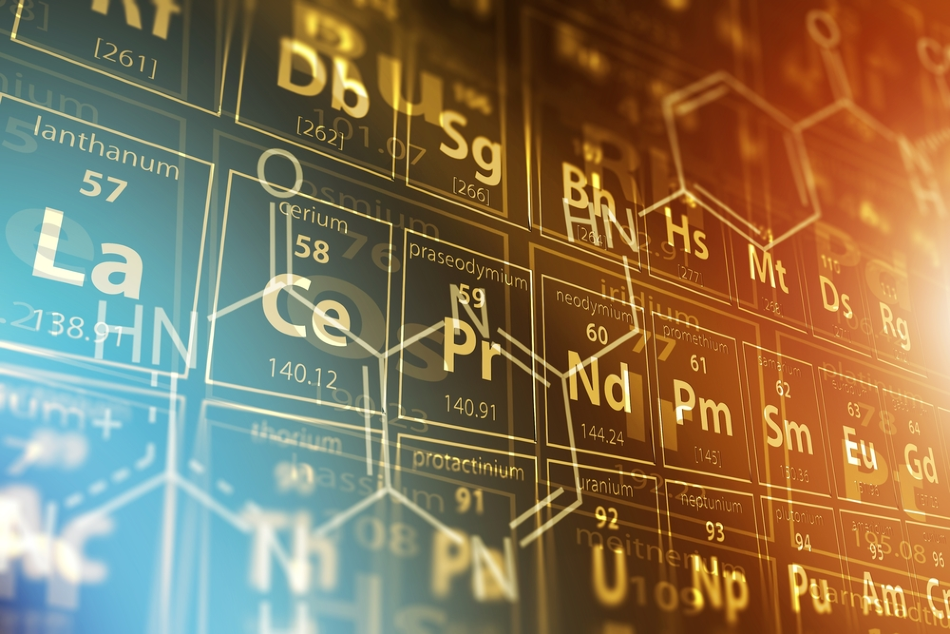
Image Credit:Shutterstock/welcomia
Can you share some of the results your study has uncovered?
There have been a lot of published results since 2014. In 2013, we acquired our first sample of californium from the US Department of Energy. That element is prepared in only the single nuclear reactor on earth - the high flux isotope reactor at Oak Ridge National Lab.
It’s a unique resource, not just for the United States, but for the planet. It is used when making these heavy elements in large enough quantities that you can use – with just slightly customized instruments that you would use in a normal chemical application - but for much smaller scales with something like californium.
What we discovered is that everybody anticipated this element would behave like the element that's directly above it in the periodic table. That element is called dysprosium, but it turned out that it didn't behave in the same way. We've studied its magnetic properties and those were also anticipated as being the same as those of dysprosium.
Dysprosium has very important magnetic properties. It's in the engine of every hybrid vehicle, so if you drive a Toyota Prius, there is dysprosium in the very strong magnet in the electric motor.
It turned out that californium didn't follow that trend. There were other people that thought it would bear similarities to other non-radioactive elements like europium and gadolinium, which also have their own applications. It turned out it didn't follow those trends either.
What we discovered is that californium actually represents a place in the actinide series, and the actinides are the bottom-most row of the periodic table - the row where elements like plutonium are. We discovered that californium actually represents a place in that series of 15 elements where chemistry abruptly changes, and that was a really big deal.
The issue is that the element that is right before it - berkelium, named after UC Berkeley - had never been interrogated on a macroscopic scale. In other words, no one had ever taken milligrams of berkelium, made new compounds, determined the structure of the molecules that are in those compounds and measured their properties.
The reason is that berkelium is radioactive. It's a short-lived element - much shorter lived than californium. Because we have to develop special techniques to interrogate californium, we were ready to do the same thing with berkelium even though no one had done that before.
There had been some limited studies on californium, but nobody had really gone after berkelium the way we did. We were able to collect data that resulted in a paper in the journal Science that was well publicized as well as a paper in the Journal of the American Chemical Society that was even more well publicized.
What we showed was, that while berkelium has some similarities with californium, there really is an abrupt break. I can't think of anything neater than telling people that chemistry fundamentally changes in part of the periodic table, because I think people have the impression when they look at that periodic table that it's immutable, that it's never going to change.
What we're telling the world - we and other groups that study the chemistry of heavy elements - is that there's actually still a lot of surprises left to discover.
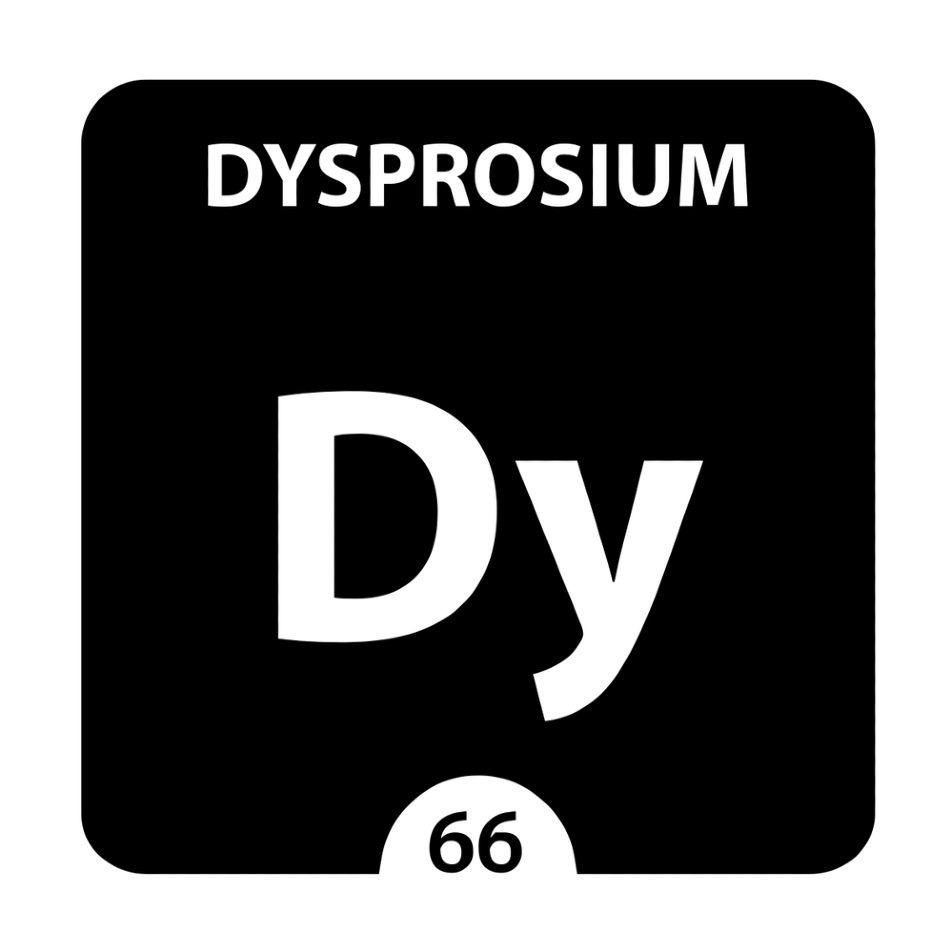
Image Credit:Shutterstock/Borka Kiss
What critical problems will you be able to solve using the results from your study?
We have designed materials that are highly resistant to radiation damage. That's important because we have a lot of excess radioactive materials that either come from defense or from power generation, and the ultimate disposal place for those is in deep geologic repositories. The US has the only one of these that's functioning right now – it’s called the Waste Isolation Pilot Plant (WIPP), which is in New Mexico.
Even though we anticipate those materials never contacting groundwater, we do have to ask the question: “What happens if they do?” We don't want groundwater somehow getting into a repository, breaking through the containers, and resulting in contaminated groundwater.
Those scenarios are very unlikely, but wouldn't it be even better if you had your radioactive elements inside of materials that were resilient? Materials that not only withstood the radiation that the plutonium or the neptunium was producing, but could also resist damage from water or oxygen. Some of the real applied things that have come out of this work is that we've designed those kinds of materials - we have viable materials for accomplishing that goal.
The second thing is that these elements, even when they're present in waste, could be recycled. They could be put into new generations of nuclear power plants, and we can fission them and get energy out of them. So rather than burying them and treating them like waste, why not treat them like a resource?
The Center for Actinide Science and Technology is an Energy Frontier Research Center funded by the Department of Energy. I direct the center, and we've developed revolutionary strategies for selectively extracting some of the elements that represent energy resources.
Let's not treat them as waste, let's treat them as a resource, because there will come a time when humans are desperate for energy resources. We've contributed to the future of taking advantage of them as a resource.
What variety of characterization tools do you use to aid your research?
When you make any new compound, you have to subject it to an array of characterization tools. You have to use a technique called x-ray diffraction, which allows you to determine the structure of that material, where all the atoms are and what atoms are stuck to what other atoms.
That information is very critical. You have to use instruments called SQUIDs (superconducting quantum interference devices), that allow you to measure magnetic properties.
You also have to measure the electronic properties, which tell you where all of the electrons are around the heavy elements. In that particular case we use a CRAIC Technologies instrument called a microspectrophotometer. It's a long name, but essentially the word spectrometer means you're measuring a spectrum of some property. In this case it's an optical spectrum, so you're looking at what colors it absorbs and what colors it transmits.
The issue that we faced is, whenever you're dealing with a radioactive material, you need to be interrogating a very small sample. In order to use the earlier technique mentioned - the x-ray diffraction - we have to grow beautiful little crystals of those samples.
What we really needed to do was collect a spectrum of those crystals. In other words, we needed to determine what colors of light that crystal absorbed and what colors it transmitted. At the time we started doing this, which is now 21 years ago, there was no capability in that area. I remember, probably 15 years ago at least, scouring the internet for anybody that made a spectrometer that could interrogate a crystal as small as the head of a pin.
There was CRAIC Technologies, who at the time were making these instruments for forensics labs. You could, for instance, take a carpet fiber from a crime scene and compare it to a carpet fiber somewhere else to determine if they were the same fiber. That was the original intent of those instruments, so you'll see them in forensics labs in the US.
We purchased one of these instruments and to my knowledge, we were the first chemistry lab to purchase one. What was really neat, is that it transformed into a relationship between my laboratory and the company providing the instrument, where they used my lab to understand the needs of chemists that required detectors with higher sensitivity and higher resolution.
It actually required many changes, so these instruments have evolved, and I have a newer instrument, not the original one I purchased. Because other people found out about my instrument, how incredibly easy it was to use and how high quality the data you obtained from it was, there's been a proliferation of these instruments throughout, not just the US, but the world.
Two of my students that are professors in China have them, many of the national labs like Los Alamos National Lab now have them and different academic institutions that saw this capability now have them.
I've been delighted to be a part of the spread of this instrument because what you don't want is people, when they're trying to publish their data, say “Look, we really can't collect this data.” We know it's important and scientific reviewers may insist on it, but it doesn't matter how much they insist. If it's not physically possible to get the data, you can't get the data.
These microspectrophotometers have had a transformative role in our research, because they allow us to produce data that may be normal in any other kind of chemical interrogation, but that we couldn't use those normal instruments for because our samples are so small. It is a workhorse instrument for us. We use it every day, and it’s actually on most of the time, every single day. It has really helped us.
Why is it different from other tools on the market? What sets it apart?
This was really an untested instrument, but that's how we discovered it. At the time I was at Auburn University and it was a few hours away at the University of Georgia, but no one had used it in a chemistry lab before.
To my knowledge, they don't have a lot of competitors, so that's the first thing that sets it apart.
People sometimes build these things from scratch - my own PhD advisor was working with a physical chemistry group that built one from scratch.
What sets the CRAIC instrument apart is that it has wonderfully integrated software. You can teach a very new graduate student how to use it in under an hour. Some of the really talented students actually get so good that they are collecting data that is of higher quality than has ever been collected before.
One of my students, Joe Sperling, collected the absorption spectrum, showing where californium absorbs colors. That spectrum he collected, because he has become a real expert in using the instrument, and it is actually the finest californium absorption spectrum ever collected. We'll publish it this year.
Californium was discovered 70 years ago, so it's not like there haven't been other opportunities to collect this data. Other people have collected californium absorption data, but it's just not like what Joe was able to obtain.
You have a wider energy window for collecting the data. It's not just the visible region of the spectrum but a lower energy region called the near IR. We did not purchase this option, but we could have collected even farther into the near IR if it had been important to us.
The instrument has excellent optics, it has a sensitive detector with high resolution, and it's a turnkey instrument - it's really easy to use.
It is not just absorption data either, because you can get absorption, transmission, reflectance and photo luminescence - this is how something glows. We collect photo luminescence data as much or even more than we collect the other kinds of optical properties. We’ve also got a second capability, which allows us to collect Raman data - which is a type of vibrational spectroscopy.
It's an all in one instrument that, with the push of a button, is up and running. You don't need to be an expert to get expert quality data. That's really what you want in an instrument, so people don't have to build their own, they can buy this fully commercialized instrument.
Many funding agencies don't want to fund instrument development. There are separate programs for doing that. So, if you're involved in synthesis and characterization, which many groups do, your funding agency does not want you wasting time trying to troubleshoot an instrument that you're building from scratch. It's much better just to buy this fully assembled system.
My instrument is also built on a ZEISS platform, and that was a very good decision for CRAIC Technologies, because it's hard to beat a ZEISS microscope. They started with something excellent and built on that - that's a great place to begin.
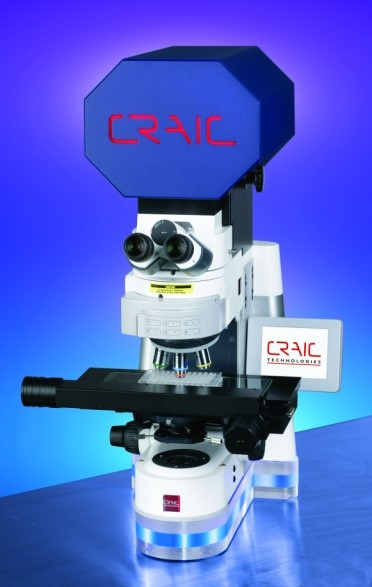
What is in store for the future of your research and potential applications?
We are trying to move deeper into the periodic table. Californium, as I said, is the last element you can have a milligram of. If you move over to einsteinium, you're down to micrograms. The first sample of einsteinium that we received was 400 nanograms, and we actually got high quality absorption and photo luminescence data from that 400 nanograms of einsteinium.
In the fall of 2019 we received our first sample of fermium, and we were able to gather data on that. What we're trying to do is go to the end. Fermium is element 100, and that is the last element where you can have a macroscopic amount. In this case it's about one picogram of atoms.
We are trying to find out, where the periodic table ends for a somewhat normal synthetic laboratory. It's somewhere between 99 and 100. There are other labs that make their own atoms, but those are very different kinds of physics labs. So that's where we're going - we're always pushing limits in our group.
About Dr. Thomas Albrecht-Schönzart 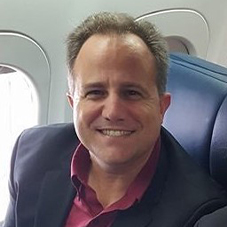
Thomas Albrecht-Schönzart is an American radiochemist specializing in the chemistry and physics of transuranium elements. He is jointly appointed as a University Distinguished Professor at the Colorado School of Mines in Golden, Colorado, and Director of the Nuclear Science & Engineering Center and Idaho National Laboratory.
Disclaimer: The views expressed here are those of the interviewee and do not necessarily represent the views of AZoM.com Limited (T/A) AZoNetwork, the owner and operator of this website. This disclaimer forms part of the Terms and Conditions of use of this website.