AZoM speaks to Ezra Lee Clark, postdoctoral research associate at Technical University Denmark about his work on electrocatalysis and the role he played in the development of the Hiden type B dual thin layer DEMS cell.
Electrocatalysis refinement is key to carbon dioxide reduction and the use of renewables in the production of plastics and fuels. A new device, the Type B DEMS cell, could herald such a refinement.
As an increasing reliance on green and renewable sources reduces electricity costs, the chemical and fuel industry continues to rely on non-renewable sources.
This reliance has urged researchers to investigate methods to utilize cheaper electricity and more common materials, like oxygen, nitrogen and carbon dioxide, to create useful products such as plastics and fuels.
One key area of research in this field is the electrochemical conversion of carbon dioxide into plastics, fuels and valuable chemicals at relatively low temperatures and in other common conditions.
This process requires a huge amount of energy, but electrocatalysis can be applied to lower the electric potential level at which reactions can occur. Understanding reaction mechanisms and identifying products can refine electrocatalysis significantly.
A new device dubbed the Hiden Type B - Dual thin layer DEMS cell was developed at Berkeley Labs in collaboration with Dr. Ezra Lee Clark from the Technical University of Denmark. It optimizes electrode configuration, electrolyte flow and membrane position to boost analysis of electrochemical reactions.
Can you explain your background and how it led you to study electric catalysis CO2 reduction?
In about 2009, I was an undergraduate. I was looking to do something with my career that would positively impact the environment, so I started working in an energy conversion laboratory.
I initially started working on reactors, CVD reactors and building nanomaterials, including coin cells, pouch cells and lithium-ion batteries that were to be used in energy conversion and storage devices. I also started building electric chemical devices to evolve hydrogen by water splitting.
Based on the work that I had done, we began publishing several papers, and from then on, I realized that research was my calling.
From there, I went to a graduate school at UC Berkeley, and I studied with professor Alex Bell, who is quite renowned in the field of heterogeneous catalysis. He has about 50 years of experience; he is one of the leaders in the catalysis field.
I was his first student to ever work on electric chemical CO2 reductions. Our project was to work on this as part of the Joint Center for Artificial Photosynthesis - a Department of Energy energy innovation hub.
The US government had put aside a significant amount of money so that Caltech, Berkeley and Stanford could all work together to try and solve this challenge. I've been working on CO2 reduction now for about ten years, but I started back in 2012 as it was the topic of my graduate dissertation.
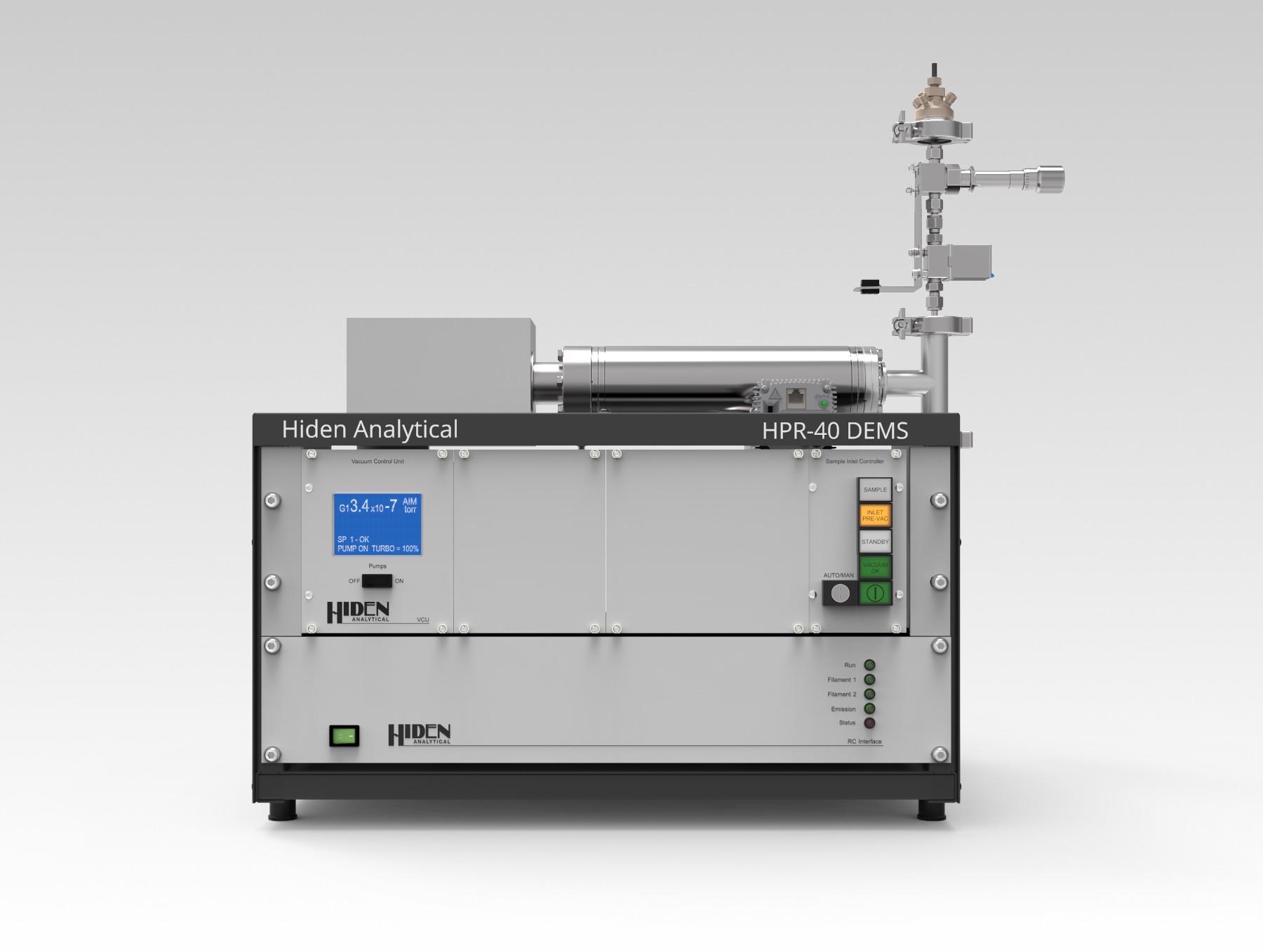
Image Credit: Hiden Analytical
What is electrocatalysis CO2 reduction in simple terms?
Electrocatalytic CO2 reduction is essentially a chemical process that allows you to take electricity and use it to convert carbon dioxide into a useful chemical directly.
It could be anything from a multi carbon alcohol, like ethanol, that could be used as a fuel, or it could be something like an aldehyde or a carboxylic acid, something that we could feed directly into the chemical industry.
It is a way to take electricity, preferably from a renewable source, like a solar cell or a wind turbine, and harness that electricity to recycle CO2.
What effect could a more widespread adoption of electrocatalysis CO2 reduction have on the environment?
If we could implement this technology at the significant sources of CO2 emissions (cement manufacturing, power plants, etc.), we could see a significant reduction in our CO2 emissions.
I hope one day that we will be able to couple this technology with the greater widespread use of renewable electricity and other strategies for capturing and mitigating CO2.
This way, we will hopefully break even and stop contributing to the increasing amounts of CO2 in the atmosphere and stabilize the environment.
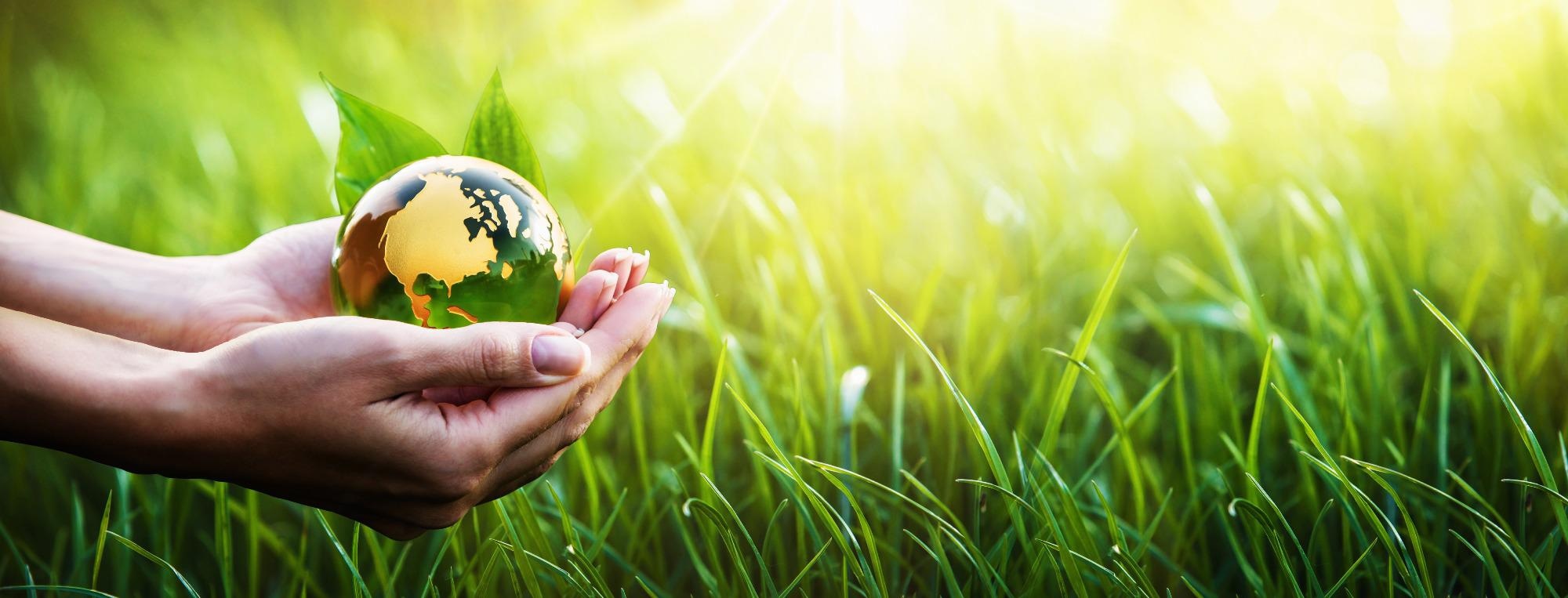
Image credit: Shutterstock.com/Pasko Maksim
Can you explain the role that you played in the development of the Hiden type B dual thin layer DEMS cell?
When I first joined Alex Bell's research group at Berkeley, our first task was to develop an analytical tool that would allow us to perform experiments in a very rapid manner and determine what kind of chemicals or fuels are being produced by this electric chemical CO2 reduction process.
The way in which to do that is to use a technique called differential electrochemical mass spectrometry, or DEMS for short. Essentially this technique takes an electrochemical cell and a mass spectrometer and interfaces the two with the pervaporation membrane.
This allows for the collection of electrochemical reaction products, such as ethanol, ethylene and aldehydes, in real time via pervaporation. This process relies on their volatility, but all of these are quite volatile.
Since these products can be collected in real-time and then analyzed with a mass spectrometer, researchers can rapidly characterize the materials or characterize the products of their electrochemical process.
As this was my first project, I was tasked with developing a way to interface this mass spectrometer and electrochemical cell in such a way that would not only allow the electrochemistry to be adequately performed but so that the mass spectrometer would generate rapid feedback so that the two could be linked together easily.
I spent about a year and a half to two years developing this technique.
Why is electrocatalysis so important now?
Electrocatalysis is so important now because we are seeing a dramatic reduction in the cost of renewable electricity. In the last 10 to 20 years, the cost of solar electricity and wind electricity has dropped dramatically – these applications are starting to become much more profitable and widespread in their implementation.
What this means is that there are now economic opportunities to not only use electricity to power our homes and our businesses but also to potentially drive chemical processes in a way that hasn't been feasible before.
Not only that, but we now know that things like our industrial activities and our use of fossil fuels are negatively impacting the environment due to the CO2 emissions that are associated.
Therefore, we not only need to develop new ways of utilizing renewable electricity, but we also need to develop ways of weaning our society off these fossil resources, and electrocatalysis is one of many tools that can be used to do this.
Electrocatalysis is perhaps one of the most elegant ways to do this because it's a single process where you're taking the electricity and these waste products from industrial processes, and you're, in one step, combining them to produce something useful while mitigating what would otherwise be discharged into the environment.
How can this device from Hiden help to improve electrocatalysis?
The device from Hiden can be used, for example, to screen catalysts in a much more rapid fashion.
For instance, if you make a new material and you want to understand what kind of products it produces as a function of voltage, using conventional techniques can take up to one week to accurately determine what kind of activity and selectivity a new material has for a given process.
Yet, using the Hiden cell that I developed, we can rapidly scan the voltage and record the reaction products in real-time by interfacing the electrochemical reactor and the mass spectrometer. We've cut down the time it takes from about one week down to just an hour or two.
The device from Hiden can also accelerate the materials discovery and thus accelerate the development of the technology and its implementation into the real world.
Hopefully, the device can also be used down the line to screen new processes. We know that electrochemical CO2 reduction works, but what else out there can we think of? What other processes could we drive with renewable electrons?
A device like this would be ideal for discovering new chemistries. It can also be used to understand the mechanisms of the reaction and how they are occurring.
With the knowledge of how the reaction occurs and through what steps the different reactants are going through to ultimately yield the useful products, we can design new catalysts to do those processes more actively and more selectively.
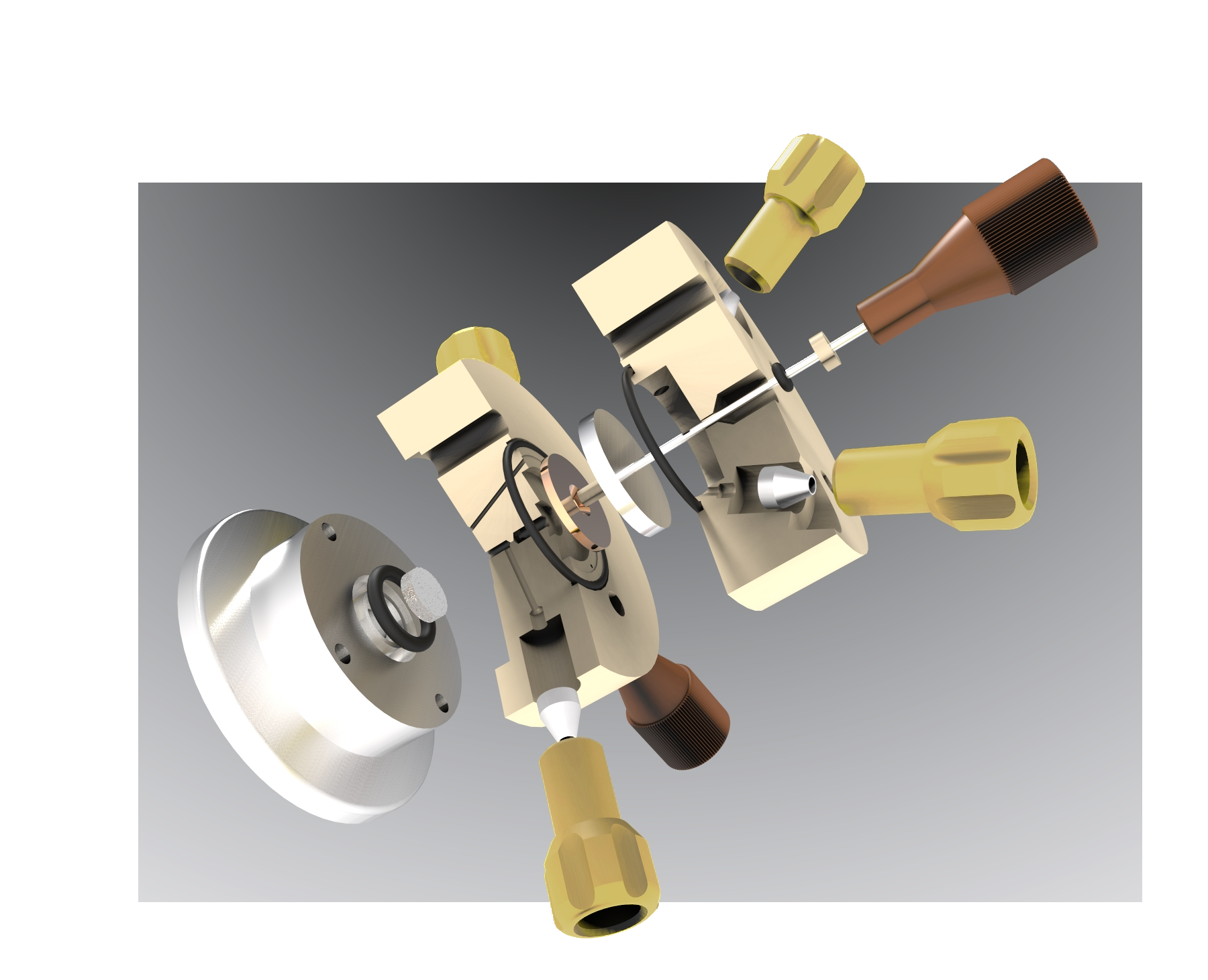
Image credit: Hiden Analytical
What sets the Type B DEMS cell apart from other similar devices?
People have been trying to make devices that unify an electrochemical cell with a mass spectrometer for probably about 40, 50 years. Yet, all of these cells have suffered from different problems, issues and challenges depending on their particular geometry.
The most common challenge was to sync the electrochemistry with the product detection to try to make it as real-time as possible; researchers brought the electrode very close - maybe a hundred microns - to the pervaporation membrane.
What this meant is that products could be collected relatively quickly. We could change the voltage in a dynamic manner and very rapidly see the impact on the product distribution with a mass spec. The issue with this was that the very thin layer of electrolyte between the electrode and the pervaporation membrane was very resistive.
This high resistance was one problem, but the other problem was that typically when you perform an electrocatalytic reaction, you want the anode or the electrode where the oxidation happens to be parallel to the cathode where the reductions occur.
The issue with this thin layer of electrolyte is it left no room for the opposite electrode. This opposite electrode, therefore, had to be somewhere else and it was usually connected somewhere off to the side. This meant that the electrodes were not parallel -there was not a parallel potential field.
This lack of parallel potential field, coupled with the high resistance, meant that the voltage varied quite significantly as a function of position on the surface of the electrode.
The goal of this technique is to know when to apply a given voltage, what is being produced and with what kind of rate is being produced. Yet, when you have a variable voltage across the surface, you don't really get the answer to those questions.
When applying a voltage, it continuously varies across the surface. If we wanted to apply a given voltage, that voltage would only be applied very close to the point where we’re sensing the potential – somewhere else down the length of the electrode there will be a different voltage.
As a result, it is very hard to establish correlations between catalyst, structure, potential or conditions in the electrolyte.
Thus, when designing the cell, I wanted to circumvent that problem while retaining the very rapid time response. At the time, and still to this day, there was no design that enabled rapid time response with parallel potential field and quantitative data.
Folks had tried to design systems that gave rapid time responses and had parallel potential fields, but they sacrificed quantifiability, and, of course, that's also very important. Therefore, this is really the only quantifiable, rapid design and is, what I would call, accurate.
The accuracy and precision came from the parallel potential field. The way we achieved this was by designing a unique type of electrode that was shaped kind of like a donut. This allowed us to flow our electrolyte in through the center of the electrode. It would then flow readily outward and be transported to the pervaporation membrane directly.
In doing this, we could bring the electrode very close to our counter electrode. This enabled us to have the parallel potential field, the rapid time response and the quantifiability as all of the reaction products that are being produced ultimately ended up in the mass spectrometer.
Are there any other unique aspects of the device that you'd like to speak about?
The device is very versatile. Aside from CO2 reduction, it can be used for any electrochemical reaction, any catalyst, and it could be used in any electrolyte conditions. What makes the device so unique is that while it has been implemented primarily for electrochemical CO2 reduction, it is quite versatile and can be used for many different things.
What do you think is next for this technology, and what improvements or breakthroughs are you personally excited about?
One of the main things that I'm interested in using this technology for in the future is the discovery of new types of chemistries, new types of chemical conversions that can be driven with electrons. I think that this sort of analytical pool is ideal for performing that kind of discovery work as it is much more rapid than conventional approaches.
Another thing that is unique about the approach is the fact that you're using mass spectrometry for product detection, and this allows you to do isotope studies, for example.
We can feed in different reactants, and if they both have carbon atoms, but we isotopically label the carbon atoms and one of those reactants, then we can get these reactants to undergo some conversion or a coupling reaction.
That product will then enter the mass spectrometer, and it will be broken apart into many different fragments. By detecting the masses of the different fragments, we can say exactly how these two molecules came together.
With that kind of information, you can then start to understand this process and couple this information with information from other techniques, like vibrational spectroscopy. In doing so, you will understand how the atoms are coming together and how they are bonding on the surface. With this knowledge, you can then start to design new catalysts and new processes.
In the next five years to ten years, I want to try to understand what other chemistries can we perform? Can we convert these standard traditional chemical processes into a greener, more sustainable version using these renewable electrons that we've generated from solar cells or wind turbines?
I think this technique will be of great help, enabling us to much more rapidly screen and test different chemistries.
Yet, one of the most underutilized aspects of this technique has really been using isotope studies to try to understand exactly how molecules are coming together. This is one of the main virtues of the DEMS technique.
Another important aspect is being able to understand the dynamic behavior of catalysts. Using conventional analytical tools, you're only able to probe what is being produced on a 10-minute timescale.
However, if we isolate the potential, for example, or make some other dynamic changes, this could yield very interesting enhancements in the catalytic rates. To do this, you need a tool that can quantify the electrochemical reaction products that are being produced on a timescale equivalent to the timescale of whatever dynamic changes you're making.
Therefore, if you want to make dynamic changes, you need an instrument that can give you real-time feedback, and that's what this technique does. In the last two to three years, we have seen some interesting work from Alex Bell's group doing this exact thing, using the tool that we developed.
Will you be working with the other developers of the type B DEMS cells again?
I will be joining the faculty at Penn State University in the US as an assistant professor in 2022. I would love to continue working with Alex, the co-inventor. We don't have any plans as of yet to continue our collaborations, but it is always a pleasure talking with Alex, and I always look forward to those discussions.
I'm also quite interested in working with Hiden Analytical down the road, as they were the ones who commercialized this technology.
I would like to work with them to continue developing this technique and improving our design, and perhaps coming up with new designs with other capabilities and unique features. We've already begun these kinds of dialogues, and I look forward to those opportunities.
If you could tell the public one thing about electrocatalysis CO2 reduction and its wider implications to capture their imaginations, what would it be?
What I would really say is that this technology provides a means of taking CO2 and renewable electricity and converting them into useful chemicals that can not only decarbonize our energy infrastructure but can also decarbonize our chemical industry.
Therefore, it has the potential to make a tremendous impact on our society, and hopefully, it captures their imagination.
About Ezra Lee Clark
Prof. Ezra L. Clark was born and raised in Louisville, Kentucky. He obtained his bachelor’s degree in chemical engineering from the University of Louisville in 2012.
During his undergraduate studies, Ezra performed research investigate the efficacy of anisotropic nanomaterials in energy conversion and storage applications under the guidance of Prof. Mahendra K. Sunkara with funding from both a Barry M. Goldwater Scholarship and a NASA Undergraduate Research Scholarship. Upon completion of his undergraduate degree, Ezra began his doctoral studies in chemical engineering at the University of California at Berkeley, ultimately earning his doctorate in 2018. During his doctoral studies, Ezra investigated the electrocatalysis of CO2 reduction under the guidance of Prof. Alexis T. Bell with funding from a NSF Graduate Research Fellowship. Ezra continued to investigate the electrocatalysis of CO2 reduction during his postdoctoral appointment at the Technical University of Denmark under the guidance of Prof. Ib Chorkendorff with funding from a Marie Curie Postdoctoral Research Fellowship. Ezra will join the faculty of the Chemical Engineering Department at the Pennsylvania State University in in 2022, where he will establish a research program investigating the electrocatalysis of chemical synthesis and conversion. To date, Ezra has authored 27 peer reviewed manuscripts, a textbook chapter, and 2 research patents which have collectively been cited over 3,600 times.
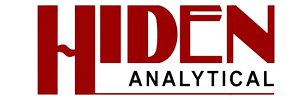
This information has been sourced, reviewed and adapted from materials provided by Hiden Analytical.
For more information on this source, please visit Hiden Analytical.
Disclaimer: The views expressed here are those of the interviewee and do not necessarily represent the views of AZoM.com Limited (T/A) AZoNetwork, the owner and operator of this website. This disclaimer forms part of the Terms and Conditions of use of this website.