Sponsored by HORIBAReviewed by Olivia FrostNov 22 2022
Semiconductors are an ever-present aspect of life as we experience it today. Application-wise, there are many factors that drive the semiconductor industry.
From displays to lighting, data communication infrastructure, power electronics, and energy, many devices start life as a wafer or wafer-like source. This article focuses on wafer characterization using a variety of different spectroscopies on one microscope platform.
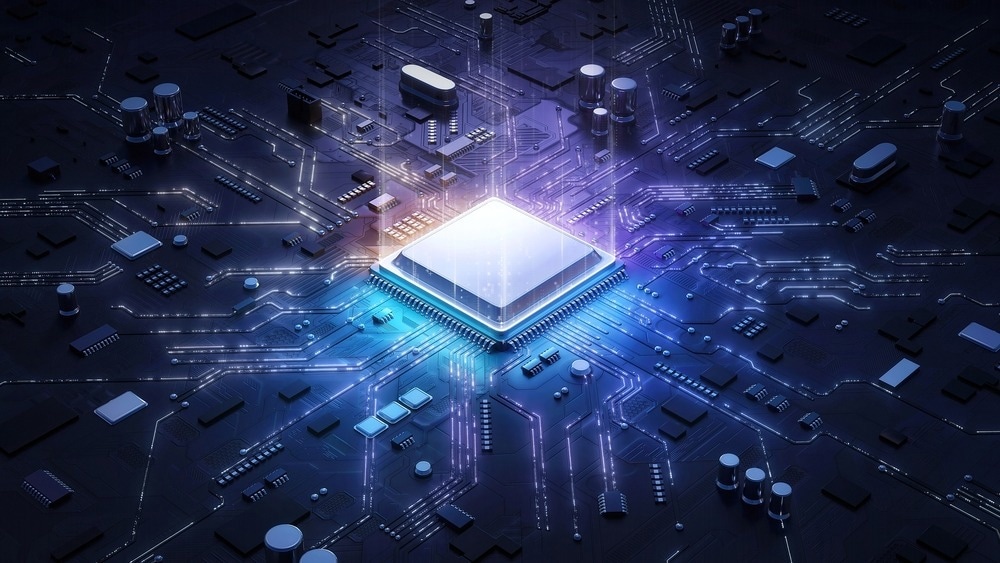
Image Credit: ShutterStock/3dartisits
Characterization Techniques for Semiconductors
Semiconductors that are often used today are highly engineered in terms of both the materials used and the designs of the device. In order to achieve high precision in the design outcomes, material design, and device fabrication, a vast number of metrologies and characterization techniques have been developed.
Amongst these, optical spectroscopic techniques hold the pride of place. This preference derives from the fact that these methods are often non-contact. Compared to other techniques, they are also comparatively fast and easy to use and usually require little to no sample preparation.
Some of the more popular techniques, such as Raman, PL, lifetime, reflectance, transmittance, electroluminescence, darkfield scattering, and photocurrent, are commonly used in today’s research and fabrication shops.
With these techniques, researchers are able to resolve issues associated with engineering a given material composition or modifying its behavior to obtain desired performance properties. It is important to note that even though several techniques can achieve a given objective, they are often not always suited to all tasks.
Even when two techniques can achieve the same aim, one is usually better at it than the other. For example, crystallinity and strength can be obtained using both Raman and PL, but Raman, due to the nature of the effect, is often more sensitive than PL and so is deemed better suited for this purpose.
Challenges and the Need for Customized Solutions When Characterizing Semiconductors
One of the biggest asks when it comes to flexible custom solutions is often multi-modality – the need to combine multiple different spectroscopies to address particular needs for an application.
One may also need to address unusually sized samples or sample conditioning requirements such as ever-increasing wafer sizes and the occasional need for low-temperature measurements at the material development stages. People have been addressing these customization needs for a long time now, mainly via DIY implementations, which leads to varying degrees of success.
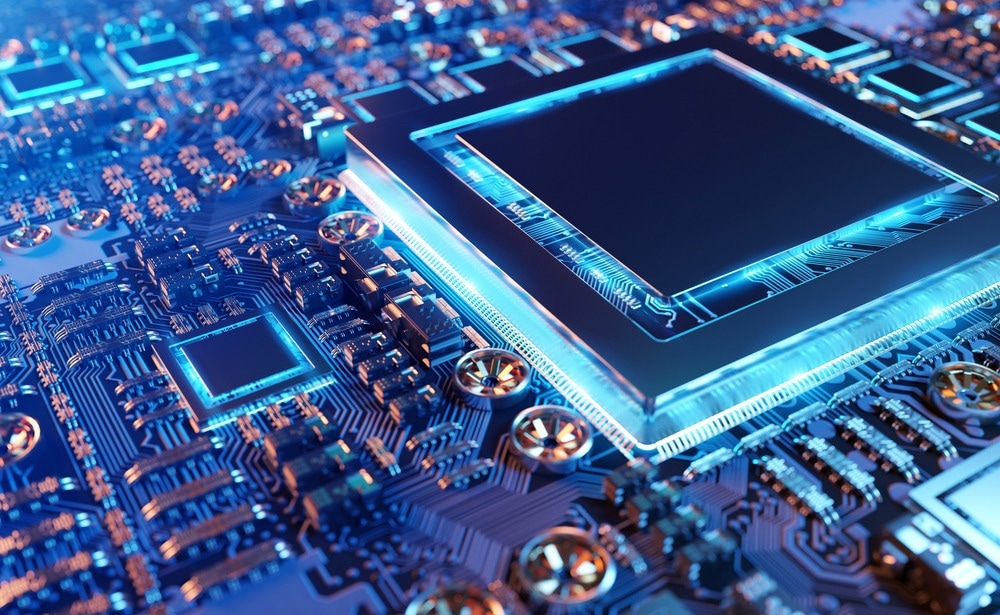
Image Credit: ShutterStock/sdecoret
Another intrinsic problem that most people face in microspectroscopy is to do with the microscopes themselves, as they are not necessarily designed for spectroscopy. Not least, though, is the fact that the implementation can be time-consuming, especially when dealing with lasers that require certain skills and knowledge to ensure that they are safely integrated.
The standard optical microscope is an imaging device typically designed to use extended light sources, such as lamps, and usually includes optical components that are not suitable for laser-based spectroscopy.
Another crucial component for spectroscopy is the filter module which can be very limiting to some demanding spectrometers such as Raman. The problem is that the filter design for standard microscopes can obscure important Raman structural modes that provide information about crystallinity.
Innovative Solutions with HORIBA
HORIBA’s solution is the SMS platform with a typical configuration that uses a standard microscope but decouples the imaging functions of the microscope from the spectroscopy function with a self-contained spectroscopy moderation. This helps to solve the problems of laser absorption or scattering optics that many DIY practitioners have had to combat when operating the microscope.
Internally, the filter design uses the principle of shallow angle design, which has great implications for optimal performance. Each filter module includes separate beam steering optics for each laser.
There are four key features that are very enabling for the SMS package. The first property is the field of design and the shallow angle filters used for spectroscopy, like Raman, which are very important when conducting structural analysis. The optics used in these modules enable very high throughput relative to a typical microscope-based system.
The second set of features is the concept of independent beam tiering for different lasers. Thirdly, there are conveniences such as polarizers when carrying out isotropic-type measurements – so there is a polarizer on the excitation and an analyzer on the emission for those types of measurements.
Finally, this platform adapts to most upright microscopes from most major manufacturers of microscopes, so it is universal in its application.
Key Application Areas for Characterizing Semiconductors
One of the key parameters for LEDs is the color or wavelength. LED color translates to a very specific spectrum, which in turn translates to the bandgap of the material involved.
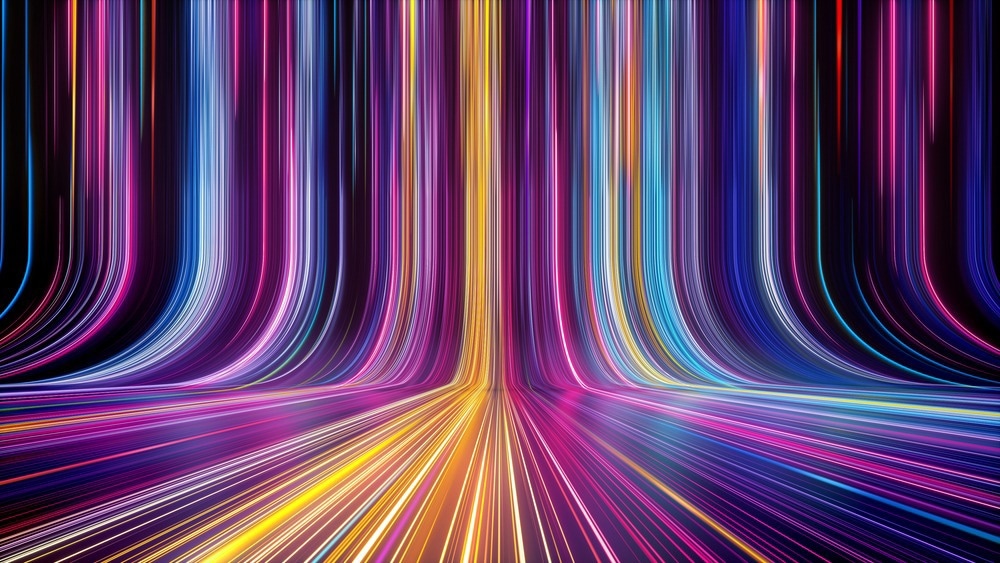
Image Credit: ShutterStock/NeoLeo
With the SMS system, the bandgaps can be characterized from the UV all the way to the Mid-IR. This is because the SMS mainly uses reflective optics, which are not prone to chromatic operations on the pathway from the wafer sample through the microscope to the spectrometer and detector.
Another important parameter and performance metric is ensuring that the position on the wafer has excellent uniformity. This determines the yield and pricing of the devices involved.
On the SMS, up to 300-millimeter wafers can be mapped with up to one-micron spatial resolution, and it features Swift – a fast mapping mode with powerful analytics of spectral parameters such as peak intensity, peak wavelength, full-width half max, and integrated power that shows the distribution of these properties. This means that the user can carry out thresholding and decision-making based on the yield.
Sometimes defects naturally occur in a fabrication process that manifests as cold spots in the PL map. For LEDs, threading these locations, which are like micro-cracks, is particularly important because these are often the dominant failure mode for LEDs.
Minimizing the occurrence or density of such defects is a fabrication imperative if a good yield and long-lived LEDs are to be realized.
With Time-Resolved PL, users can identify defects and also understand the dynamics involved in recombination. An important consideration for yield for LEDs is the power consumption budget, which can relate back to things like defect concentration. On the SMS, this can be characterized with a high spatial resolution using electroluminescence.
Other techniques apply to PV and are similarly applicable to LEDs. Photovoltaic applications rely on optimized photoelectric charge generation and moving these charges efficiently through a load or battery for energy storage. This ladder performance requirement means that carrier dynamics is a very important consideration for PV applications.
In addition to point measurements for Time-Resolved PL, the SMS system can also do FLIM, which is Time-Resolved PL imaging. Such measurements can clearly indicate where charges are moving efficiently and where they are not and what is responsible for that behavior. These kinds of measurements offer a lot of insight because of the ability to capture dynamics.
Regarding Raman, there are difficulties addressing the occasional external contaminants that may result from the application process or material handling. When these contaminants are on the micro-scale, it can be a challenge to identify them or what they are.
Compared to many other micro PL mapping tools, the SMS is a true micro spectroscopy tool capable of resolving down to one micron.
With a technique like Raman spectroscopy, SMS can measure the Raman spectral fingerprint of contaminants. Furthermore, because the SMS is a modular addition to the standard microscope, it can benefit from other microscope imaging modalities, such as darkfield, to enhance the resolution for such measurements.
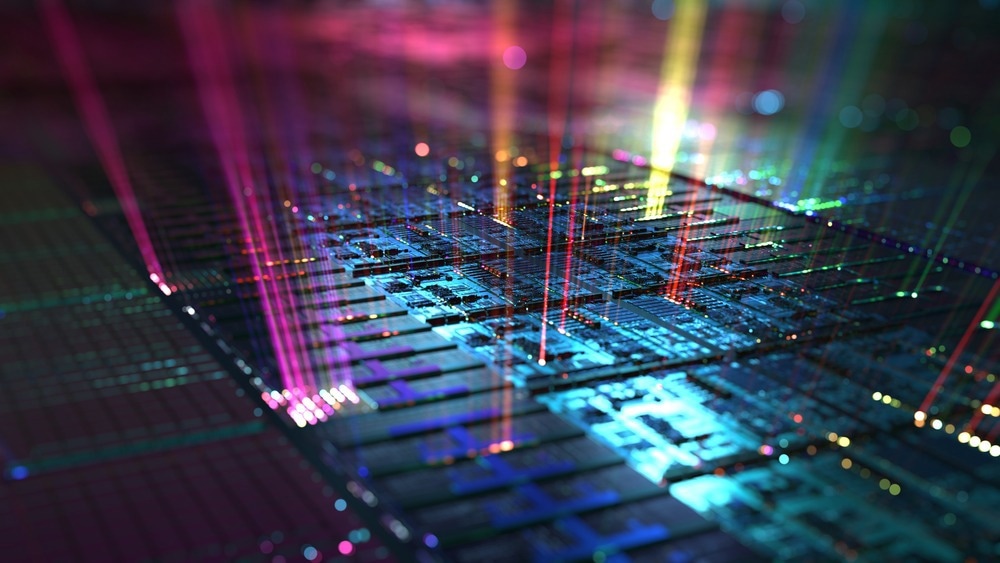
Image Credit: ShutterStock/Quardia
With optional Raman libraries built into the SMS software, the user not only gets to measure the spectrum of the contaminant but can also identify what the contaminant is.
Finally, we can use photocurrent to get a sense of the real-life performance of PV devices. Charge generation and movement is an important figure of merit for PV devices, and because it measures a current, it gives an excellent indication of charge generation and transport across the PV device. On the SMS, this measurement can be done with discrete lasers.
Increasing Applicability of the SMS System
In certain scenarios, measurements need to be conducted across different systems because the systems in use cannot be fully integrated, as is the case with SMS.
The challenge becomes twofold: how can a degree of integration be achieved between the two systems, and secondly, how can the user reliably and quickly locate and measure potentially soft macro-size objects across these different platforms?
While SCM and SMS are two different platforms, they are partially integrated, share the same spectrometer and detector, and run on the same software. This two-in-one system helps with affordability while streamlining training and ease of use by using the same software.
With the SMS, users have access to up to eight different optical spectroscopies, while the SCM offers high specialty resolved CL, which is particularly helpful in the case of high bandgaps semiconductors.
Even though these two systems share some common components, they are nevertheless two different systems, and measuring across them still means taking a sample from one system to another. When this is a sub-micron sample, it can be quite difficult and time-consuming just to locate the sample.
Researchers at HORIBA have been working to address this issue with a patented nanoGPS tag called navYX package. The technology involves a geotag that is attached to the sample substrate and can be imaged with the camera on each system.
Using this product allows for a coordinate transformation between two different systems so that sub-micro size objects can be quickly and reliably located between the two systems.
In conclusion, the SMS is a modular, versatile, and affordable platform that adds one or more spectrometers to any standard microscope.
It is a multi-modal platform because it combines multiple complementary spectroscopies on the same platform, all without compromising on performance, either in spectroscopy or the native imaging functions of the microscope.

This information has been sourced, reviewed and adapted from materials provided by HORIBA.
For more information on this source, please visit HORIBA.