Gold nanoparticles (AuNPs) have been utilized since before the scientific revolution. The earliest known use was in the stained glass of the Lycurgus cup, thought to be preserved from the fourth century, A.D.2
Gold nanoparticles have also often been used in stained glass windows, as shown in Figure 1. Gold particles dispersed in the glass result in a deep red color that can be observed when light is shone through it.
A nanoparticle (NP) is a particle with at least one dimension between 1 and 100 nm in size. Metallic NPs are now widely utilized in scientific research due to their interesting and tuneable physical, chemical, and optical properties compared to their bulk metal counterparts.
This is especially true for AuNPs as they have optical properties that are easily manipulated and related to their chemical environment and physical state.1 This allows them to be precisely engineered for various applications.
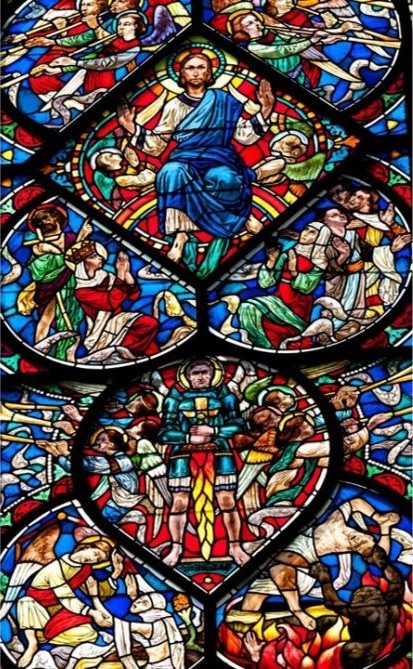
Figure 1. Gold nanoparticles are responsible for the red tint in stained glass windows and pieces of art. Image Credit: Edinburgh Instruments
Colloidal AuNPs are valuable for colorimetric and optical detection applications due to their absorbing and scattering light efficiency. They may also be tuned to display optical activity at different wavelengths based on their chemical and physical characteristics.
They can support a localized surface plasmon resonance (LSPR) as a result of a displacement and coherent oscillation of the conduction electrons on the NP surface at a specific frequency following excitation by incident light.3
The LSPR is a highly valuable parameter for optical sensing as its frequency is heavily dependent on multiple factors, such as NP morphology, NP size, interparticle distance, and the refractive index of the surrounding dielectric medium.
All these factors may be designed to change in response to an external variable in a detection assay.
Traditionally, the optical properties of AuNPs are probed via UV-Vis spectroscopy as it is an excellent method for characterizing a sample’s response to light over a great wavelength range. The LSPR is visible as a distinct peak on the absorption spectrum of AuNPs.4
This article presents a study of the optical properties of AuNPs using UV-Vis spectroscopy. The LSPR of spherical colloidal AuNPs is first examined as a function of particle diameter.
Subsequently, the chemical sensing potential of AuNPs is shown through tracking the LSPR during an induced aggregation experiment.
Materials and Methods
The DS5 UV-Vis Spectrophotometer from Edinburgh Instruments was employed to conduct UV-Vis spectroscopic measurements. Gold nanoparticle colloid solutions of different sizes were acquired from BBI Solutions, while sodium chloride was acquired from Sigma Aldrich.
Sodium chloride was dissolved in distilled water and a small aliquot was subsequently added to a solution of AuNPs to induce aggregation.
To perform the UV-Vis absorption measurements, a 1.5 mL aliquot of the sample was placed in a 1 cm path length cuvette in the sample light path, while distilled water was placed in a cuvette in the reference light path.
Samples were analyzed in the range of 400 nm to 1000 nm with a scan speed of 800 nm/min and a read interval of 1 nm.
Results and Discussion
To examine the relationship between the LSPR and particle diameter, various spherical AuNP samples that had particle diameters in the range of 10 to 80 nm were characterized via UV-Vis spectroscopy, as shown in Figure 2.
Spherical AuNPs that are stable in solution are simple to identify using UV-Vis spectroscopy due to a sharp absorption band being exhibited, with a λmax typically between 500 nm and 600 nm, as displayed in Figure 2.
The results also demonstrate that the LSPR wavelength, based on the λmax values in the normalized absorbance spectra, redshifts as the size of the NPs increases. This change of the LSPR to a longer wavelength is caused by the electrons oscillating over a greater area and, as a result, at a lower frequency.
The LSPR redshift with increasing particle size is beneficial to the design of optical nanosensors for biological purposes as these applications need near-infrared (NIR) excitation sources. A change in color is also observed with increasing particle size and the corresponding absorption spectra explain this occurrence.
The smaller NPs absorb strongly in the green part of the visible spectrum, leading to a rich ruby-red color that makes them well-suited for colorimetric assays, including lateral flow testing devices.
As the NP size increases and the LSPR redshifts, more red light is absorbed, and the colloidal solution displays a purple hue.
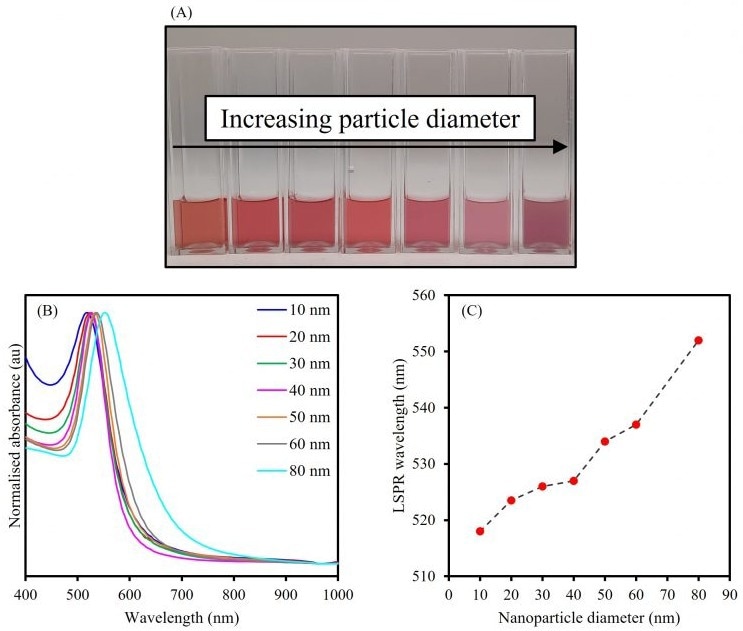
Figure 2. The effect of increasing particle diameter on the LSPR of spherical AuNPs. Image Credit: Edinburgh Instruments
UV-Vis spectroscopy, and by extension the LSPR, may also be employed to monitor changes in the stability of colloidal AuNPs. A loss of stability causes individual NPs to aggregate into clusters that change their shape, size, and interparticle distance.
The controlled stimulation of aggregation is useful and can be utilized in colorimetric and LSPR assays, as well as in nanosensor design for NIR biological applications.5 However, when aggregation is not controlled, it may lead to the irreversible dissolution of NPs.
To show the effect of aggregation on LSPR, 20 nm AuNPs were mixed with salt solutions of various concentrations and subsequently analyzed via UV-Vis spectroscopy, as shown in Figure 3.
AuNPs are typically prepared using a method that involves a gold salt being reduced with sodium citrate, leading to the particles being capped by citrate anions. The net negative charge on the surface of each AuNP greatly contributes to the colloid’s stability.
The addition of salt results in this negative charge being masked, and the electrostatic interactions that initially prevented aggregation of the AuNPs are removed, significantly decreasing the stability of the colloid.
Without an aggregating agent, the 20 nm AuNPs exhibit an LSPR at 524 nm and are a deep red color. When a low concentration of the salt solution is mixed with the NPs to induce a controlled aggregation, a color change is observed from deep red to pink, but stability is preserved.
This solution’s absorbance spectrum exhibits distinct changes from the bare AuNPs. Despite the λmax remaining consistent with the bare NPs, the absorbance of the LSPR peak is dampened and an increased response is observed in the NIR range from 600 nm to 1000 nm.
The dampening of the original LSPR band demonstrates that the number of stable 20 nm NPs in the colloid has reduced because of aggregation.
Additionally, the increased optical activity in the NIR range suggests that aggregation has occurred because it indicates that the free electrons on the surface of each NP are now delocalized and shared among other NPs within aggregated clusters, resonating at a lower frequency than the original LSPR.
A greater concentration of salt being mixed with the bare AuNPs results in these effects being even greater, with the solution becoming purple in color before the aggregates come out of the solution.
The λmax of the original LSPR peak redshifts from 524 nm to 532 nm, which is indicative of the delocalization of surface electrons due to the formation of NP clusters.
The susceptibility of citrate-capped AuNPs to salt-induced aggregation can be an issue, particularly when the AuNPs are introduced to biological media. As a result, it is common for encapsulation in polyethylene glycol or silica to be carried out beforehand to increase their stability and biocompatibility.
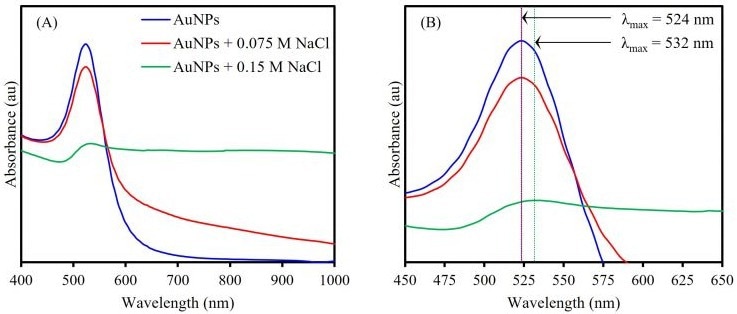
Figure 3. UV-Vis absorption spectra (A) showing salt-induced aggregation’s effect on AuNP LSPR and (B) comparing LSPR of the bare AuNP, controlled AuNP aggregate, and uncontrolled AuNP aggregate solutions. Image Credit: Edinburgh Instruments
Conclusion
The DS5 Dual Beam UV-Vis Spectrophotometer from Edinburgh Instruments was employed for the characterization of the optical properties of various AuNP samples.
Gold nanoparticles are increasingly popular in a wide variety of sensing applications, and UV-Vis spectroscopy is an excellent method for guaranteeing sample stability, as well as fine-tuning the physical, optical, and chemical properties of the samples.
References and Further Reading
- R. Sardar et al., Gold Nanoparticles: Past, Present, and Future, Langmuir, 2009, 25, 13840–13851.
- The British Museum, Drinking-Cup, https://www.britishmuseum.org/collection/object/H_1958-1202-1 (accessed January 2023).
- A. Willets et al., Localized Surface Plasmon Resonance Spectroscopy and Sensing, Annu. Rev. Phys. Chem., 2007, 58, 267–297.
- W. Haiss et al., Determination of Size and Concentration of Gold Nanoparticles from UV-Vis Spectra, Anal. Chem., 2007, 79, 4215–4221.
- S. Unser et al., Localized Surface Plasmon Resonance Biosensing: Current Challenges and Approaches, Sensors (Basel), 2015, 15, 15684–15716
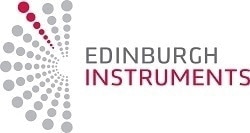
This information has been sourced, reviewed and adapted from materials provided by Edinburgh Instruments.
For more information on this source, please visit Edinburgh Instruments.