Identifying the presence of unwanted liquids within pressurized gas pipelines is one of the key challenges faced by the petrochemical industry as their presence raises safety concerns. The liquid sample analysis can only be performed remotely and by means of a non-intrusive approach. Determining the contaminant to let the liquid source to be identified is one of the critical steps in tackling this problem.
The need to making accurate measurements of liquid samples approximately 1mm in depth from a distance in excess of 2m and negating the effect of the surrounding gas on the identification method limits the determination technique to some form of laser-induced spectroscopy.
IS-Instruments, together with GL group, National Grid UK, and IMA Ltd., has created a new class of Raman spectrometer to determine the trace levels of these unwanted liquids in gas pipelines. Raman measurements were performed within the GL gas flow loop, a facility that emulates the Gas National Transmission System.
In this work, lab trails were performed to measure four different liquids from a distance of 2m, and then a field trail was carried out to measure the liquids in a pressurized gas flow loop in the field. This article discusses the use of the IS-Instruments High throughput spectrometer in the successful completion of these measurements.
IS-Instruments High Throughput Raman Spectrometer
It is a difficult task to make Raman measurements from a distance of several meters because Raman spectra are very weak. The signal strength is eventually a function of the laser power telescope area product:
.jpg)
Image Credit: IS-Instruments
Where, Lph is the number of laser photons emitted per second,
A is the receiving aperture area,
α is the Raman scattering cross section,
N is the number of molecules intercepted,
OE is the instrument optical transmission,
QE is the detector quantum efficiency,
t is the time of integration,
T is the transmission through the atmosphere, and R is the distance to the target source.
The use of a hot tap and the insertion of an optical window are required to perform measurements within the pipeline. A 3” NB hot tap was considered for the test set-up, presenting a working aperture of <75mm.
As this application demands spectral measurements with a resolution of <5cm-1, most commercially available spectrometers are not suitable for this application due to their inadequate etendue, or throughput, characteristics, which would result in more light loss and poor SNR measurements.
Based on its range of HES spectrometers, IS-Instruments in collaboration with IMA Ltd., has built a high-performance spectrometer for application in gas pipelines. This challenging application leverages the high etendue provided by the spectrometer, thereby making the Raman measurements possible.
The spectrometer was equipped with a cooled low noise detector to determine the Raman signals of liquids from a distance of roughly 2.5m. The complete instrument was developed inside a weatherproof, ATEX approved enclosure for field application, as shown in Figure 1. The system was connected to a 1mm diameter, 0.22 Na fiber without any slit.
.jpg)
Figure 1. Prototype spectrometer used on a test pipeline.
Image Credit: IS-Instruments
Specifications of IS-Instruments High Throughput Raman Spectrometer
The specifications of the IS- Instruments high throughput Raman spectrometer are listed in the following table:
Table 1. Instrument specifications.
Parameter |
Specification |
Operating wavelength |
785nm |
Laser power |
500mW |
Laser divergence |
4mrad |
Resolution |
4cm-1 |
Range |
150–2700cm-1 |
Detector QE |
70%(at 785nm) |
Dark noise |
0.004e/s |
Receiving aperture |
70mm (diameter) |
Experiment Procedure and Results
Liquid samples of xylene, methanol, ethylene glycol (MEG), and triethylene glycol (TEG) were measured using the instrument. For the site trials, a gas flow loop test facility was fitted with a modified spool piece to allow filling of a section of the pipe with the appropriate liquid.
The approximate length of the gas flow loop filled with natural gas is 30m. The liquid was fed into the spool piece and allowed to settle prior to making measurements. Xylene was the first liquid analyzed. The returned measurement from a 20mm of xylene is illustrated in Figure 2, showing the spectra from 0-2500cm-1.
.jpg)
Figure 2. Xylene spectra: Black line represents 20mm of xylene.
Image Credit: IS-Instruments
Figure 2 shows multiple strong peaks. The corresponding Raman spectrum from a 2 mm liquid level is depicted in Figure 3, which is also showing multiple peaks as observed in Figure 2. This illustrates the superior sensitivity of the complete system despite the low liquid level. The broad feature observed at around 1400cm-1 is owing to the presence of residue compressor oil at the pipe bottom, which returns a broad fluorescent signature. A spectrum of a completely clean pipe is also depicted in Figure 3 for completeness.
.jpg)
Figure 3. Xylene Raman spectra, red line represents <2mm of xylene; blue line represents no liquid present.
Image Credit: IS-Instruments
The Raman spectra observed for methanol with an estimated depth of slightly over 10mm are illustrated in Figure 4, returning a clear Raman spectrum. The results are in good agreement with lab measurements.
.jpg)
Figure 4. Methanol spectra: Black line represent 10mm of methanol, blue line is the background level.
Image Credit: IS-Instruments
The Raman spectra observed for MEG are shown in Figure 5, displaying four strong peaks with a chain of weaker bands throughout the range being analyzed.
.jpg)
Figure 5. <20mm of ethylene glycol (MEG); triethylene glycol (TEG).
Image Credit: IS-Instruments
TEG was the final chemical analyzed for an estimated level of <10mm and the resulting spectra are demonstrated in Figure 6. Although there is a similarity in the appearance of the MEG and TEG spectra, differences can be clearly observed, especially in the structure of the peaks at around 800cm-1. The noise of the TEG spectrum is higher due to weaker return signal caused by the presence of a lower amount of liquid in the pipe.
.jpg)
Figure 6. Raman spectra of triethylene glycol (TEG).
Image Credit: IS-Instruments
Conclusion
The results clearly demonstrate the ability of the IS-Instruments high throughput Raman spectrometer to measure liquid samples in pressurized pipelines. The system is all set to be deployed in an extended field trial on a working pipeline.
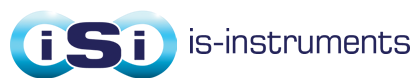
This information has been sourced, reviewed and adapted from materials provided by IS-Instruments.
For more information on this source, please visit IS-Instruments.