Nov 11 2013
Volatile organic compounds (VOCs) are carbon-containing compounds that evaporate easily at normal temperatures. They are typically generated from petroleum and gasoline products, plastics, paints, solvents, cleaners and degreasers, inks, dyes, refrigerants and pesticides.
Other sources are the disinfecting agents used to control microbial contaminants in drinking water. Disinfecting agents react with naturally occurring organic matter in the source water to produce VOCs known as trihalomethanes (THMs).
Health Concerns over VOCs
VOCs vary considerably in their toxic effects. Depending on their levels, VOCs may be harmful to the central nervous system, the kidneys or the liver; cause skin irritation upon contact; and irritate the mucous membranes if inhaled. Some VOCs, like the trihalomethanes, are known or suspected carcinogens.
Analytical Methods for the Analysis of VOCs in Water
Over the last decade, numerous analytical methods have been developed to identify and measure VOCs in ground and surface water, wastewater, and drinking water. Currently, the most widely used method for VOC analysis is purge-and trap sample concentration combined with gas chromatography - mass spectrometry (GC-MS). P&T is capable of separating and concentrating most VOCs in water samples. When combined with GC-MS, sub-ppb levels can be measured.
The Need for High Purity Water for VOC analysis by P&T GC-MS
The highest purity water can be obtained by combining several purification technologies as shown in Figure 1. The ultrapure water produced is suitable for use in GC-MS analysis of VOCs since it is free of any compounds that could interfere with the analysis.
.jpg)
Figure 1. A complete chain of water purification technologies that efficiently removes contaminants in tap water to produce ultrapure water with the lowest levels of VOCs
Reverse osmosis (RO) removes over 95% of a wide range of contaminants that includes organic molecules. Electrodeionization (EDI), which uses continuously regenerated ion exchange resins, further reduces ionic contamination, including charged organics. The purified water is polished by the Q-Gard® and Quantum® cartridges, using synthetic activated carbon to further reduce organic contaminants, and ion exchange resins to further remove
Experimental Details
Part 1 of the experiment was designed to trace the levels of 58 VOCs in the various stages of a water purification chain. Table 1 lists the VOCs that were analyzed. In part 2, the ability of the VOC-Pak™ cartridge to remove various VOCs was tested by carrying out a challenge test using 25 VOCs. The last part of the experiment analyzed the VOC content in bottled water commonly used as reference water in VOC analyses.
- Injection mode: Purge and Trap. An Eclipse 4660 Purge-and-Trap Sample Concentrator (O I Analytical Corporation, College Station, TX, USA) was used. The trap consisted of Tenax®, silica gel, and charcoal. Sample volume was 25 mL.
- Separation: Gas Chromatography. An Agilent® 6890N Network GC system (Santa Clara, CA, USA) was used with an Agilent J&W DB-624 column (30 m x 0.25 mm x 1.4 pm) under constant pressure mode (7.93 psi).
- Detection: Mass spectrometry. An Agilent 5975B inert XL EI-MSD was used in the scanning mode (mass range: m/z 35 - 300).
- Analytical standards were from ULTRA Scientific (N. Kingstown, RI, USA).
- Water purification system: Tap water fed an Elix® 10 system to produce pure water. The pure water was fed to a Milli-Q® Advantage A10® system to produce ultrapure water. It contained two cartridges: a Q-Gard® T1 and a Quantum® TEX, which consist of synthetic activated carbon and ion exchange resins.
Table 1. List of VOCs analyzed In the various stages of a water purification chain. These compounds are regulated by different agencies around the world (World Health Organization, USA, EU, Japan, China, Canada, Australia).
1,2-dibromo-3-chloropropane |
bromobenzene |
1,4-dichlorobenzene |
bromochloromethane |
1,2-dichloropropane |
bromomethane |
1,2-dibromoethan |
n-butylbenzene |
benzene |
sec-butylbenzene |
ethylbenzene |
fert-butylbenzene |
styrene |
chloroethane |
toluene |
2-chlorotoluene |
o-xylene |
4-chlorotoluene |
m-xylene |
dibromomethane |
p-xylene |
1,3-dichlorobenzene |
1,1-dichloroethene |
1,1-dichloroethene |
vinyl chloride |
1,3-dichloropropane |
carbon tetrachloride |
2,2-dichloropropane |
chlorobenzene |
1,1-dichloropropene |
1,2-dichlorobenzene |
cis-1,3-dichloropropene |
1,2-dichloroethane |
trans-1,3-dichloropropene |
cis-1,2-dichloroethene |
hexachlorobutadiene |
trans-1,2-dichloroethene |
isopropylbenzene |
methylene chloride |
4-isopropyltoluene |
tetrachloroethene |
naphthalene |
1,2,3-trichloropropane |
n-propylbenzene |
1,1,1-trichloroethane |
1,1,1,2-tetrachloroethane |
1,1,2-trichloroethane |
1,1,2,2-tetrachloroethane |
trichloroethene |
1,2,3-trichlorobenzene |
bromodichloromethane |
1,2-trichlorobenzene |
bromoform |
trichloromonofluoromethane |
chloroform |
1,2,4-trimethylbenzene |
Purge-and-Trap (P&T) GC-MS analysis of VOCs is routinely carried out in many environmental laboratories to evaluate water quality. Because of the very low detection limits of this method, it is important that the water used to prepare blanks, standards, and dilute samples (when necessary) be free of the VOCs to be analyzed.
An experiment was designed to track the levels of 58 VOCs in the various stages of a water purification chain as shown in Figure 1. Water samples were taken from tap, after pretreatment (RO and EDI), and the ultrapure water that was delivered through a point-of-use (POU) cartridge that is specific for VOC removal (the VOC-Pak™).
An example of a mass chromatogram of tap water from Saint-Quentin-en-Yvelines, France is shown in Figure 2. Five VOCs were identified: chloroform, bromodichloromethane, tetrachloroethylene, dibromochloromethane, and bromoform.
.jpg)
Figure 2. Mass chromatogram of tap water
To quantify the VOCs, calibration curves were prepared from standard solutions. The calibration curves in this work were well below the maximum contaminant levels (MCL) imposed by regulatory agencies in various countries. The levels at which the VOCs were detected in the samples were very low, so the concentrations of the standards used to make the calibration curve had to be within this low range.
Out of the 58 VOCs that were tested, only five were detected in tap water at levels between 0.07 - 18 ppb1 (Table 2.): chloroform, bromodichloromethane, tetrachloroethene, dibromochloromethane, and bromoform.
Table 2. VOC concentrations in tap water, purified water after RO and EDI, after further polishing with activated carbon and ion exchange resins (Q-Gard®), UV photooxidation, and after purification by the VOC-Pak™ cartridge.
VOC |
MCLa (ppb) |
Detection Limit (ppb) |
Concentration (ppb) |
|
Tapc |
After RO and EDI |
After Q-Gard® |
After UV |
After Quantum® + VOC-Pak™ |
Chloroform |
80b |
0.05 |
7.61 |
3.34 |
0.06 |
0.06 |
ND |
Bromodichloromethane |
80b |
0.05 |
14.92 |
1.40 |
ND |
ND |
ND |
Tetrachloroethene |
5 |
0.05 |
0.07 |
ND |
ND |
ND |
ND |
Dibromochloromethane |
80b |
0.10 |
17.75 |
0.33 |
ND |
ND |
ND |
Bromoform |
80b |
0.50 |
4.42 |
ND |
ND |
ND |
ND |
a MCL values from US Environmental Protection Agency
b The MCL is for total trihalomethanes (chloroform, bromoform, bromodichloromethane, dibromochloromethane).
c Tap water is from St.-Quentin-en-Yvelines, France.
ND = Not detected
After the tap water was pretreated by RO and EDI, tetrachloroethene and bromoform were no longer detected, while the other three VOCs were still present although in significantly reduced concentrations (Table 2). This was expected, since low molecular weight neutral organics like chloroform are not efficiently retained by the RO membrane. The EDI module is only efficient at removing ions, and not uncharged organics like VOCs.
The pretreated water was then polished using synthetic activated carbon, UV photooxidation, ion exchange resins, and a VOC-Pak™ cartridge. Adsorption onto activated carbon is a widely used process to remove trihalomethanes (THMs) such as the ones detected in the tap water (chloroform, bromodichloromethane, tetrachloroethene, dibromochloromethane, bromoform). In any case, bromodichloromethane and dibromochloromethane were no longer detected after passing through the Q-Gard® cartridge (Table 2).
A challenge test was carried out to test the capacity of the VOC-Pak™ cartridge in removing various VOCs. Ultrapure water (resistivity of 18.2 MΩ.cm at 25°C, TOC ≤ 5 ppb) that was spiked with various VOCs was made to pass through a VOC-Pak™.
The concentrations of the VOCs before and after passing 300 L of the spiked water through the VOC-Pak™ are shown in Table 3.
VOC tested |
Detection limit (ppb) |
Concentration before VOCPak (ppb) |
Concentration after VOC-Pak™ (ppb) |
benzene |
0.05 |
0.97 |
<0.05 |
bromodichloromethane |
0.05 |
1.56 |
<0.05 |
bromoform |
0.50 |
1.58 |
<0.50 |
carbon tetrachloride |
0.05 |
1.06 |
<0.05 |
chlorobenzene |
0.05 |
0.94 |
<0.05 |
chloroform |
0.05 |
1.07 |
<0.05 |
dibromochloromethane |
0.10 |
1.01 |
<0.10 |
1,2-dichlorobenzene |
0.05 |
1.01 |
<0.05 |
1,4-dichlorobenzene |
0.05 |
1.53 |
<0.05 |
1,2-dichloroethane |
0.05 |
1.08 |
<0.05 |
1,1-dichloroethene |
0.05 |
0.87 |
<0.05 |
cis-1,2-dichloroethene |
0.05 |
0.95 |
<0.05 |
trans-1,2-dichloroethene |
0.05 |
1.53 |
<0.05 |
1,2-dichloropropane |
0.05 |
0.86 |
<0.05 |
cis-1,3-dichloropropene |
0.05 |
0.97 |
<0.04 |
trans-1,3-dichloropropene |
0.05 |
0.91 |
<0.05 |
ethylbenzene |
0.05 |
1.09 |
<0.05 |
tetrachloroethene |
0.05 |
1.44 |
<0.05 |
toluene |
0.10 |
0.96 |
<0.10 |
1,1,1-trichloroethane |
0.10 |
0.94 |
<0.10 |
1,1,2-trichloroethane |
0.05 |
0.93 |
<0.05 |
trichloroethene |
0.05 |
1.02 |
<0.05 |
o-xylene |
0.05 |
0.79 |
<0.05 |
m-xylene |
0.05 |
1.02 |
<0.05 |
p-xylene |
0.05 |
0.94 |
<0.05 |
Bottled water that is commonly used as reference water in VOC analyses was analyzed and compared with ultrapure water purified by a VOC-Pak™. Figure 3 is an overlay of the mass chromatograms of the two types of water compared.
As shown in Table 3, the VOC-Pak™ cartridge is able to reduce the VOCs to levels that are below the limits of detection of the experimental method.
Conclusion
In conclusion, the highest purity water for VOC analysis can be obtained by combining several purification technologies and a point-of-use (POU) cartridge (VOC-Pak™) containing activated carbon specific for the removal of VOCs. The ultrapure water produced is suitable for use in the measurement of VOCs by sensitive methods such P&T GC-MS since it is free of any compounds that could interfere with the analysis.
About EMD Millipore - Lab Water Business Unit
Water is the most commonly used solvent in laboratories and constitutes often more than 99% of the mass of solutions used in experimentations. The quality of water used in the lab is therefore critical for the success of the tests performed.
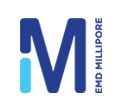
This information has been sourced, reviewed and adapted from materials provided by EMD Millipore - Lab Water Business Unit.
For more information on this source, please visit EMD Millipore - Lab Water Business Unit.